ABSTRACT
Antimicrobial resistance (AMR) has emerged as a high priority global problem in recent decades. Its severity lies in the critically increasing number of pathogenic bacteria that carry resistance genes to previously common antibiotics (ATB), making them a health threat. The emergence of resistance is a consequence of the long-term misuse of ATB in human medicine and veterinary practice (with the most significant contribution from developing countries). In 2017, the UN warned that the issue is not limited to these areas and that the environment can also be a significant reservoir and vector for the spread of AMR. The issue has been included in the “One Health” initiative, which is based on a collaborative approach to combat AMR across the health, agriculture, and environment sectors. AMR enters the aquatic environment in the form of resistant bacterial strains (ARB) or resistance genes (ARG) shed by patients through municipal wastewater treatment plants (WWTPs), runoff, or agricultural waste.
In our study, screening was performed for the occurrence of ATB resistance to selected ATBs (cefuroxime, cefotaxime, cefepime, gentamicin, sulfamethoxazole/trimethoprim, fosfomycin, nitrofurantoin and meropenem) in indicator bacteria Escherichia coli (E. coli) isolated from surface water and wastewater in influent and effluent of a wastewater treatment plant. A culture disk diffusion method was used to detect resistance. E. coli with proven resistance was detected in almost 100 % of the samples tested, with the exception of resistance to nitrofurantoin and meropenem in samples of the category above the WWTP effluent. The highest proportions of resistant E. coli were found for gentamicin in all categories. Multi-resistant strains and strains producing extended-spectrum beta-lactamases (ESBLs) were also observed.
The detection of high numbers of resistant or multi-resistant E. coli strains in and downstream of treated effluents indicates the importance of the aquatic environment in the spread of AMR, which may be a consequence of the suggested transfer of resistance between bacterial strains in WWTP. The screening findings point to the need for detailed study of AMR in the environment, which is essential for success in efforts to reduce the current health threats posed by ATB resistance in the Czech Republic and worldwide.
INTRODUCTION
Antimicrobial resistance (AMR) is the ability of bacteria to resist the effect of antibiotics (ATBs), i.e. substances that can kill them or stop their growth. The natural property of every organism, including bacteria, is to survive and reproduce. If contact with ATB prevents them from doing so, they will try to find a way to avoid their negative effect. This is how mutations and genetic transfers occur, which cause the initially sensitive bacteria to become partially or completely resistant to the action of ATB. The main cause is excessive contact of bacteria with ATB caused by their incorrect or inappropriate use and the occurrence of ATB in the environment. The consequence is the fact that there are dangerous resistant bacteria in the world today, for which neither standard nor reserve ATBs work. Currently, we can only defend against them with higher doses or other types of ATB which, however, are in limited quantities; this can mean a greater burden on the body and more side effects for patients. At the same time, there is a possibility that the bacterium will find a way to defeat ATB; over 35,000 people are reported to die in the EU each year related to AMR [1, 2].
Contributing to the spread of AMR is the excretion of ATB into wastewater (up to 80 %) and the overuse of ATB in the agricultural sector, where, until 2006, preventive administration of ATB to farm animals to promote growth was practiced (and in some non-EU countries it is still being practiced). The emergence of resistant bacteria in the ATB-contaminated environment is a source of AMR that has not yet been fully explored. In the clinical sector, the use of broad-spectrum ATBs that act against a wide spectrum of bacteria, underdosing of recommended therapeutic doses that cause bacteria to adapt to low ATB levels, and inconsistent diagnosis of the causative agent (e.g. viral infections treated by ATB) contribute to AMR.
ATB effect of is also complicated by the ability of some bacteria, including E. coli, to produce extended spectrum beta-lactamases (ESBL), which hydrolyse frequently used ATB (including penicillins and cephalosporins).
Clinical and veterinary medicine at the European and global level has been studying AMR and the effects of its spread intensively. In 2019, the WHO ranked AMR among the ten most significant health threats; in 2022, the European Commission, together with EU member states, designated AMR as one of the three priority health threats [3]. Adopted in June 2023, the European Council’s recommendation on strengthening EU measures to combat antimicrobial resistance within the framework of the “One Health” approach [4] now contains specific goals that each member state should achieve by 2030. For the Czech Republic, the goals are listed in the Strategy of the National Antibiotic Programme of the Czech Republic for 2024–2030:
- reducing total ATB consumption by 9 % (compared to 2019), whereby at least 65 % of ATB used in all EU states should be basic, narrow-spectrum ATB;
- reducing the overall incidence of bloodstream infections caused by ATB-resistant bacteria; for methicillin-resistant Staphylococcus aureus, the incidence should decrease by 6 %, for third-generation cephalosporin-resistant E. coli by 5 %, and for carbapenem-resistant Klebsiella pneumoniae by 2 %.
AMR in the environment was not a priority concern until recently. Findings demonstrating its importance were accepted in 2017 in the UN Frontiers 2017 study [5–7]. Professor W. Gaze pointed out that ATB release is an overlooked problem, but one that could be key to the development of resistant strains, and sparked a commitment to tackle AMR across sectors, resulting in the “One Health” initiative. The risk lies in the fact that the majority of ATB in non-metabolized form together with resistant bacteria (ARB) gets into water and soil, where it meets environmental bacteria and creates conditions for the mutual exchange of genetic information. Environmental conditions and other contaminants (heavy metals, disinfectants, etc.) also contribute to the transmission, which can increase selection pressure and thus the potential for the emergence of a large number of new resistances. Pathogenic bacteria with clinically relevant genes originating from the environment have been found [7]. So far, both resistant and multi-resistant bacteria (i.e. those that carry resistance to more than three ATB groups) have been found in all types of water, including groundwater. Contamination with resistant bacteria or resistance genes is risky for sources of drinking water and surface water used for bathing, where it can be transmitted to the human body via the faecal-oral route. Food chain AMR contamination can occur with irrigation water, aquaculture, and the application of sewage sludge and farmyard manure to agricultural land [8]. The mechanisms of possible aquatic environment contamination by AMR are shown in Fig. 1.
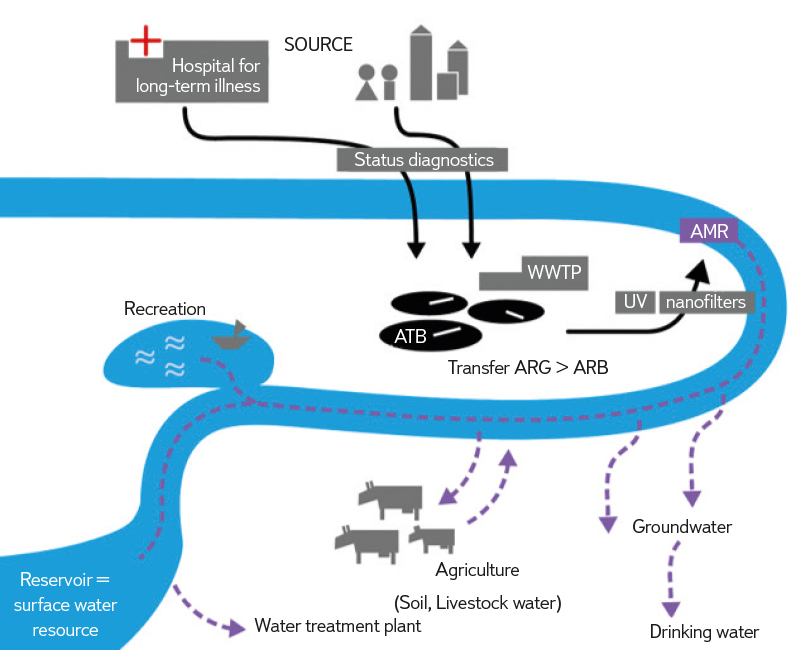
Fig. 1. Mechanisms of potential spread of AMR in the water environment
The aquatic environment is contaminated with resistant bacteria primarily through wastewater treatment plants (WWTPs), which are considered hot spots for the spread of AMR in the aquatic environment. Together with ATB, ARBs enter WWTP from human digestive and excretory systems and are present here in varying degrees of metabolism, depending on their stability in the aquatic environment. Despite the high efficiency of existing treatment technologies, which reach values of around 99 % when removing microbial pollution, a large amount of ARB and ARG is released into the recipient. A large amount of ATB which cannot be broken down by current technologies is also present in treated municipal wastewater and wastewater from the production of pharmaceuticals discharged into rivers, together with little-known products of their decomposition. To supplement information on the AMR occurrence in the population connected to individual WWTPs [4], data is also used which is obtained during monitoring of raw wastewater based on WES principle (Wastewater and Environmental Surveillance).
Knowledge of the current state of AMR occurrence in the Czech Republic is at a very low level; following the activities of other EU countries, it is necessary to contribute to its expansion in order to obtain data for effective protection of human health and the environment.
In the Czech Republic, there is currently no systematic monitoring of watercourses with regard to AMR. Information on the status can only be derived from the research activities of several scientific teams that deal with this issue from different perspectives (e.g. University of Chemistry and Technology, Prague; Pardubice University; Veterinary University, Brno; National Institute of Public Health, Prague). Interest in the AMR issue is supported by the revised Urban Wastewater Treatment Directive 271/91/EEC, which will enter into force at the end of 2024. Within this Directive, a number of changes are expected to help improve the quality of surface water and reduce the health risks associated with its use. The monitoring of substances that can affect human health should be gradually introduced, including, in addition to AMR, the direct monitoring of viruses, PFAS (perfluorinated and polyfluorinated alkyl substances) and microplastics. In the future, the issue should also be included in the Water Framework Directive 2000/60/EC.
Our goal was to obtain initial information about AMR occurrence in surface and wastewater in the Czech Republic. The screening was aimed at detecting the occurrence of antibiotic resistance to selected ATBs in the indicator bacterium E. coli, isolated from surface and wastewater at WWTP influent and effluent using the disk diffusion method.
METHODS
Sampling
To compare the WWTP influence, the locations of surface water from main watercourses above and below the effluent of municipal wastewater from large urban areas with uniform sewage, and surface water samples from smaller watercourses flowing into the Vltava were selected. At the same time, wastewater samples were analysed at the influent and effluent of these WWTPs. The samples were taken continuously in 2022–2024 and were classified into the categories ABOVE (13 samples from watercourses above large municipal WWTPs), BELOW (53 samples from watercourses below the effluent of treated wastewater from large municipal WWTPs at a distance of 500 m to 10 km), INFLUENT (19 samples from influents to the WWTP after rough mechanical pretreatment), EFFLUENT (26 samples of treated wastewater on effluent from WWTPs with different treatment technologies), and STREAM (20 samples from the Vltava tributaries of different water bearing, into which smaller WWTPs and other effluents are discharged). A total of 131 samples were included in the study. Samples were taken in the standard sampling method for microbiological analysis.
Procedure for E. coli isolation and determination of sensitivity to antibiotics by disk diffusion method
In the samples, E. coli bacteria were determined by cultivation on mFC agar [9]. From each sample, in the optimal case, four different E. coli strains were selected and isolated, for which AMR was determined by the disk diffusion method. A pure bacterial culture grown overnight on a solid non-selective medium (Trypton Yeast Extract Agar) was suspended in physiological solution to a turbidity level of 0.5 ± 0.1 according to the McFarland turbidity scale, i.e. 1–2 × 108 cells/ml. The suspension was spread evenly on plates with Mueller-Hinton agar, on which disks containing ATB of different concentrations were subsequently placed using an applicator (Tab. 1). After 18 ± 2 hours of incubation at 36 ± 2 °C, the inhibition zones of individual ATBs were read (breakpoint means of inhibition zones were adopted from the EUCAST tables [10]), see Fig. 2. ATBs and their concentrations were selected based on information on the occurrence of resistance in clinical areas, the use of ATB in the Czech Republic, and the properties of ATB in the aquatic environment so as to cover as many ATB groups as possible (source: NRL for ATB SZÚ, EUCAST [10]).
Tab. 1. List of antibiotics used and their concentration in the discs
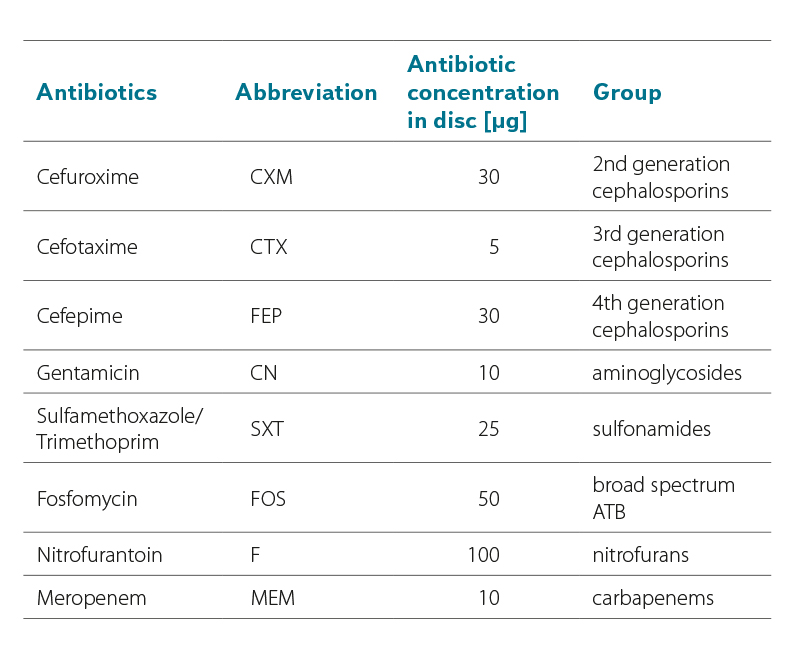
Fig. 2. Inhibition zones of the tested E. coli strain, example of a sensitive (obvious inhibition zone around the antibiotics disc) and resistant strain (small or no inhibition zone around the antibiotics disc); the size of inhibition zones is given in EUCAST
E. coli determination with production of extended-spectrum beta-lactamases
The determination of E. coli resistance to selected ATBs was complemented by the detection of the production of extended-spectrum beta-lactamases (ESBL).
A selected sample volume (usually 1–100 ml) was filtered through a sterile nitrocellulose membrane filter with a porosity of 0.45 µm, which was then placed on a TBX agar (Tryptone Bile × Glucuronide agar) plate supplemented with cefotaxime (4 µg/ml). TBX agar without ATB was used to determine the total number of E. coli in the water sample. Cultivation took place in an incubator at a temperature of 36 ± 1 °C for 21 ± 3 hours. From each sample, four presumptive colonies of ESBL-positive E. coli strains were subjected to two tests – CDT (Combination Disk Diffusion Test) and DDST (Double Disk Synergy Test) according to the procedure for performing and interpreting the results [11], see Fig. 3. ESBL detection uses inhibition of ATB hydrolysis by clavulanic acid. For CDT, cephalosporin disks containing cefotaxime and ceftazidime and combined cefotaxime/clavulanic acid and ceftazidime/clavulanic acid disks are used. Four disks (two cephalosporins and two combination disks) are used per isolate. The interpretation of the CDT test results (Fig. 3) is based on the reading of the size of the inhibition zones of each cephalosporin separately compared to the combination of the cephalosporin and clavulanic acid. For DDST, cephalosporin disks and a clavulanic disk are used. The principle is to use cephalosporin disks next to a clavulanic disk with a distance of 20 mm from the centre. After incubation, the interaction between individual cephalosporins and clavulanic acid is monitored (Fig. 3).
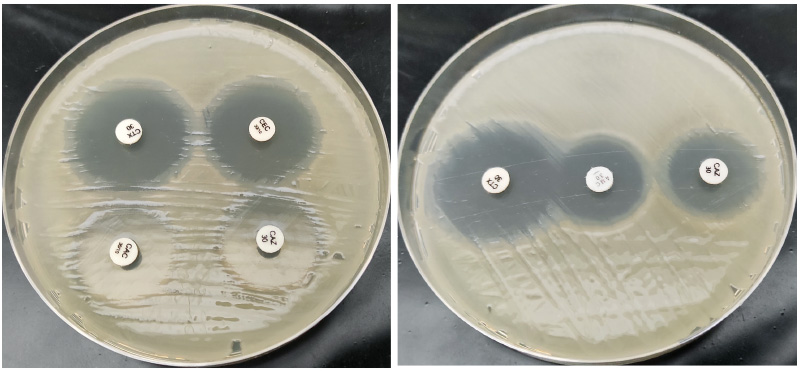
Fig. 3. Confirmation of ESBL on the E. coli isolates by CDT and DDST tests (above: CDT test, below: DDST test)
Evaluation of results
The samples were divided into five categories to evaluate the indicative occurrence of AMR in surface and wastewater. Samples taken at influents (INFLUENT) and effluents (EFFLUENT) from large WWTPs, in watercourses above (ABOVE) and below (BELOW) the effluent of treated wastewater from WWTPs and in smaller watercourses (STREAM) where smaller WWTPs are located were compared. The obtained results were evaluated within individual categories and processed into a graph. The assessment was made for the “relative percentage of strains with proven resistance” to individual ATBs. This was obtained by adding the actually tested proportion of strains to the total number of E. coli in the sample.
The accuracy of the results is affected by the relatively low proportion of tested strains (0.00004–0.19 %) due to high microbial load of surface and wastewater samples. The proportion of ESBL-positive and multi-resistant strains was evaluated separately (i.e. strains with simultaneous resistance to at least three groups of ATBs, with 3rd and 4th generation cephalosporins considered as one group).
RESULTS
During 2022–2024, 131 water samples from five categories were tested. The numbers of samples in individual categories are shown in Tabs. 2 and 3. Tab. 2 also shows the relative percentage shares of E. coli strains with proven antibiotic resistance to the tested ATB in individual categories; Tab. 3 shows the number of samples with proven antibiotic resistance to the tested ATB in individual categories. The results are shown in Fig. 4.
Tab. 2. Relative proportion of E. coli strains with proven AMR in each category
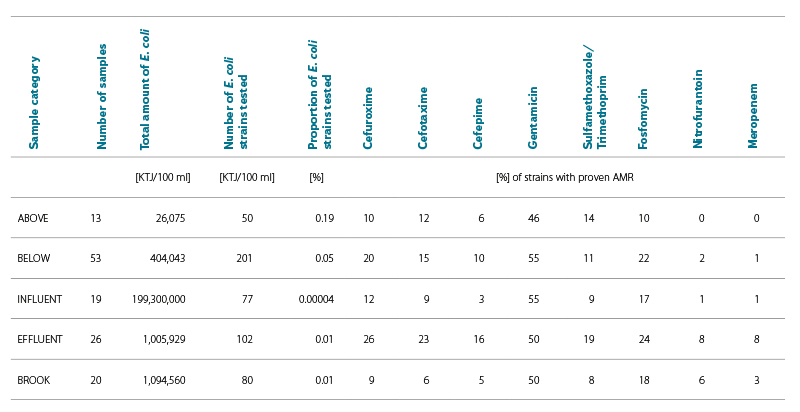
Tab. 3. Numbers of samples in each category with demonstrated AMR in E. coli
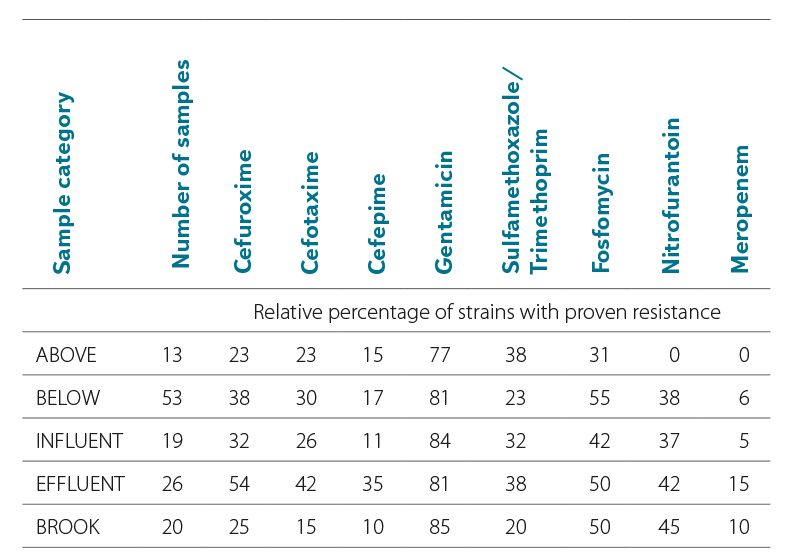
Fig. 4. Relative proportion of E. coli strains with proven AMR in each category
Most samples were tested from the BELOW category, which included wastewater recipients at different distances (500 m to 10 km) from their effluents. In this category, 201 E. coli strains were tested out of a total of more than 400,000 detected. Resistance to all tested ATBs was found; compared to the other categories, there was a high proportion of fosfomycin-resistant strains (22 %), in a similar proportion to the INFLUENT and EFFLUENT category (17–24 %). The most common was resistance to gentamicin (55 %), the least common, as in the other categories, was resistance to meropenem (1 %).
Lower proportions of antibiotic-resistant strains were found in the ABOVE category, where the profiles of larger watercourses above the WWTP effluent were included. This category served as a control comparison of the status above versus the status below the effluent of large WWTPs, which are considered significant AMR sources. However, even in this “control” category, E. coli with resistance to six of the eight tested ATBs were found. However, no sample showed resistance to nitrofurantoin and meropenem.
Other significant categories for comparison were INFUNENT and EFFLUENT from the WWTP. Despite a significant reduction in the number of E. coli in the effluents due to good treatment efficiency, resistance to all tested ATBs was found in both categories. The share of both resistant strains and positive samples was unexpectedly higher in the EFFLUENT category. The exception was resistance to gentamicin, which was similarly high in both categories (50–55 %).
The most significant increase in the proportion of ARBs occurred with cefepime, nitrofurantoin, and meropenem.
In the STREAM category, where samples of different Vltava tributaries were included, into which smaller WWTPs are discharged, resistance to all tested ATBs was also demonstrated. The proportions of resistant E. coli and samples were lower, similarly to the ABOVE category.
The largest proportion of resistant E. coli and samples was clearly identified for gentamicin (46–55 % of strains), while the least represented was resistance to meropenem and nitrofurantoin (0–8 % of strains). Resistance to second generation cephalosporins was detected in 9–26 % of E. coli strains; it was also significant in third and fourth generation cephalosporins (3–23 % of strains), see Fig. 5.
Fig. 5. Relative proportion of occurred resistance to individual antibiotics (incl. Multiple resistance; in %)
Many E. coli strains showed multiple resistance (Fig. 6). The occurrence of resistance to three to five ATB groups was most common in the BELOW and EFFLUENT category. In the EFFLUENT category, resistance to six and seven ATB groups was also detected.
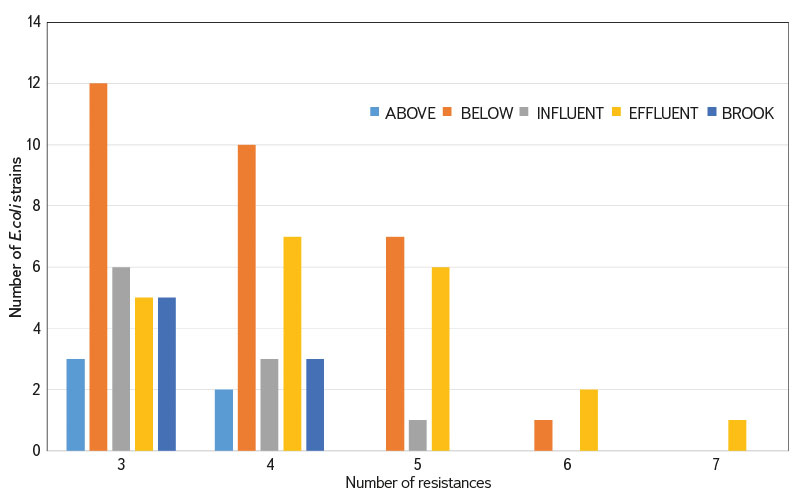
Fig. 6. Number of E. coli strains with proven resistance to 3–7 groups of antibiotics
In part of the samples processed for the international action within the framework of EIONET WG on AMR in surface waters, E. coli isolates were tentatively tested for the production of extended-spectrum beta-lactamases. Five samples (25 isolates) in the BELOW category and eight samples (33 isolates) in the EFFLUENT category were processed in this way. The proportion of ESBL strains was higher in samples from WWTP effluents (0.2–3.6 %); the occurrence of ESBL-positive E. coli was also demonstrated in the recipients (BELOW category): see Tab. 4.
Tab. 4. Ratio of samples with proven ESBL in E. coli
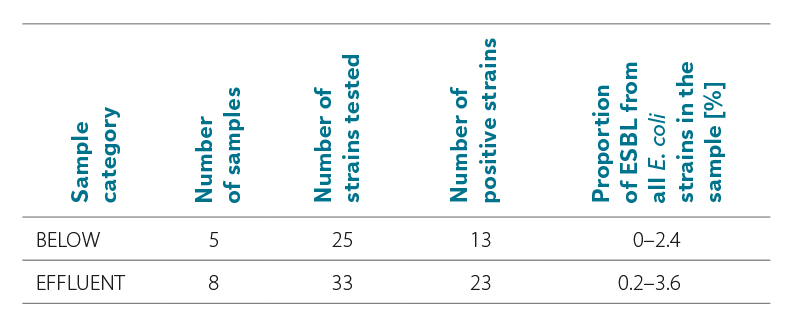
DISCUSSION
The role of the environment in the AMR development and spread is receiving increasing attention. Based on current knowledge, it is not possible to predict the intensity of AMR occurrence from any other data (e.g. ATB residues, nutrients); therefore, it is necessary to monitor AMR directly, i.e. with ARB or ARG. Among ARBs, AMR is most often observed in E. coli isolates [12, 13]. In our study, E. coli isolates from surface and wastewater were tested for the presence of AMR. ATBs to which significant resistance is currently documented in clinical sphere and which cause difficulties in the treatment of serious infections were mainly chosen for testing.
Our results confirm that the AMR occurrence in the aquatic environment is not unique; on the contrary, in the vast majority of analysed samples (95 %), E. coli isolates were found to be resistant to at least one ATB. The most common was resistance to gentamicin, fosfomycin, and beta-lactam ATB. Gentamicin is an ATB used against serious infections. It is often administered in combination with other beta-lactam ATBs and is mainly used in surgery [14]. It is known for the frequent occurrence of resistance to it [15]. Fosfomycin is an ATB used to treat uncomplicated urinary tract infections. It is also used against already resistant strains of bacteria [16]. The least frequent was the occurrence of resistance to meropenem (a carbapenem). These ATBs are among the less commonly used, for the treatment of serious infections caused by multi-resistant strains of enterobacteria and non-fermenting gram-negative rods. Resistance to carbapenems is therefore a very serious issue from a clinical and epidemiological point of view [17]. The results of this study are consistent with our earlier data [18]. Various studies document that the proportion of resistant enterobacteria from wastewater, including E. coli, can range from less than 1 % to more than 20 %, especially for penicillins, cephalosporins, quinolones, and tetracyclines [12, 13, 19]. A research team from the USA addressed a similar topic [20]. The authors describe the AMR occurrence in Salmonella, E. coli, and enterococci in surface and wastewater. Their results showed the AMR occurrence in 9.6 % of Salmonella isolates, 6.5 % of E. coli isolates, and 6.8 % of enterococci isolates. AMR for tetracycline and ampicillin was most often detected in E. coli isolates. Similar results were observed in other works, such as [21, 22]. In our study, 8 % (for meropenem) to 55 % (for gentamicin) of strains with proven resistance were detected. Overall, 60 % of all strains showed resistance to at least one ATB. Samples from WWTP effluents contained a significantly higher proportion of resistant strains, especially to cefepime, nitrofurantoin, and meropenem, which may be a dangerous consequence of suitable conditions for resistance transfer in WWTP technologies. Multi-resistant strains can be found in the aquatic environment as well. These were isolated mainly from surface water below the sewage effluent and in the effluents from the WWTP. The assumption that WWTPs act as hot spots for AMR spread of is thus confirmed.
ESBL was detected in the majority of E. coli isolates, with a share of up to 3.6 % of all E. coli strains in the sample. These findings are not an exception; they were confirmed, for example, by the above-mentioned authors [20]. Resistance to beta-lactam ATBs, particularly through extended-spectrum beta-lactamases and carbapanemases, is increasing and is a significant worldwide problem.
Current wastewater treatment technologies are not able to remove AMR sufficiently. The solution could be wastewater treatment directly at the source (hospitals, nursing homes, homes for the elderly, abattoirs, etc.), i.e. before the pollution reaches a WWTP (https://www.niva.no/en/projects/hotmats). Attention is often focused on testing advanced procedures (e.g. ozonation, use of UV), nano and ultrafiltration, but also nature based solutions (e.g. root cleaning plants) [23–25].
Importation of resistant bacteria from third world countries also appears to be a significant problem, undesirably widening the spectrum of resistances with which human medicine can no longer work. In recent years, AMR determination in the aquatic environment has been directed towards the use of molecular-biological methods based on PCR. There are many studies that deal with ARG determination [26–28]. Attention is paid to ARGs with common occurrence in the aquatic environment, but also to those that are clinically significant, such as genes encoding extended-spectrum beta-lactamases and carbapanemases [26, 28]. Both mentioned approaches have their advantages and disadvantages, but in order to obtain the most comprehensive information, it is most appropriate to combine them [19].
The issue of AMR in the aquatic environment has been receiving a lot of attention abroad for a long time, an example being France with the AMR-Env network (https://amr-promise.fr/amr-env/). In the Czech Republic, until recently, activities in the field of AMR have been directed mainly at clinical and veterinary medicine, with the environment being largely neglected. The issue of AMR in the aquatic environment has been addressed by several research teams, for example a team from the University of Chemistry and Technology, Prague [29], the Faculty of Chemical Technology at the University of Pardubice [30], the team of doc. Dolejská at Brno University of Veterinary Sciences [31], and the National Institute of Public Health (EU-WISH project). Our team at TGM WRI deals with the study of AMR in the aquatic environment, especially within the framework of projects No. SS02030008 “Centre for Environmental Research: Waste and Circular Management and Environmental Safety” and No. SS02030027 “Water systems and water management in the Czech Republic under conditions of climate change”. It is also involved in several initiatives such as EIONET WG on AMR in surface waters, AMR One Health Network, CZEPAR, Central Coordination Group of the National Antibiotic Programme.
CONCLUSION
The aim of our study was to examine AMR occurrence in different types of aquatic environments with regard to its possible sources, which could be municipal WWTPs. Resistance was determined using the disk diffusion method on E. coli isolates, detected by default as an indicator of faecal water pollution. The relative proportion of E. coli strains with proven resistance to some of the eight ATBs, classified into seven groups, was determined in the samples. Samples taken between 2022 and 2024 were evaluated within five categories of differently polluted surface water and wastewater.
E. coli with proven resistance was determined in almost 100 % of the tested samples, with the exception of resistance to nitrofurantoin and meropenem in samples of the ABOVE category. The highest proportions of resistant E. coli were found for gentamicin in all categories.
The most AMR positive strains and samples were detected in WWTP effluents, simultaneously with a significant increase in numbers when compared to raw wastewater samples in WWTP influents. The cause may be the dangerous transfer of resistance between bacterial strains in WWTP conditions. Positive AMR results were also found in samples from large watercourses above the WWTP effluent, while below the WWTP effluent there was a relatively significant increase in the occurrence of resistant E. coli, which confirms the assumption that treated wastewater from the WWTP is the source of AMR in watercourses. The category of samples from smaller tributaries of the Vltava, on which WWTPs are located, was at the medium level of AMR load, which shows that even these smaller streams need to be monitored. The proven occurrence of multi-resistant strains in effluents from WWTPs and below their effluent into recipients, together with the occurrence of E. coli producing extended-spectrum beta-lactamases, is alarming.
Our study showed that the problem of AMR in the environment needs to be given increased attention; so far, it has been neglected, not only in the Czech Republic. In key categories of water samples in the Czech Republic, the presented study showed an almost 100 % occurrence of E. coli bacteria resistant to at least one of the tested ATBs, including resistance to fourth generation cephalosporins. Detection of high numbers of resistant or multi-resistant E. coli strains in treated wastewater and below their effluents shows the importance of the aquatic environment in AMR spread and the necessity of its detailed study in efforts to reduce the current health threats posed by antibiotic resistance in the Czech Republic and around the world.
Acknowledgements
The article was created with the support of institutional resources of the MoE and projects No. SS02030008 “Centre for Environmental Research: Waste and Circular Management and Environmental Safety” and No. SS02030027 “Water systems and water management in the Czech Republic under conditions of climate change” of the Technology Agency of the Czech Republic. The authors thank the WWTP operators for their helpful cooperation in providing wastewater samples.
The Czech version of this article was peer-reviewed, the English version was translated from the Czech original by Environmental Translation Ltd.