ABSTRACT
As part of the research activities of the Hydrology Department of CHMI Ostrava, field investigations and measurements are being carried out in several catchments to verify the outputs of GIS tools, empirical formulas, and mathematical models focused on surface runoff, fluvial erosion, and sediment transport. The main emphasis is placed on the influence of deforestation and land use changes on rainfall-runoff relations and fluvial erosion, especially within the framework of the NAZV “DEFOREST” and “CLIMCFOR” projects, in which CHMI collaborates with the Forestry and Game Research Institute (VÚLHM), the Bishopric of Ostrava-Opava, and Water Management Development, and Construction joint stock Company (VRV). The presented article deals with the possibilities of analysing fluvial processes and disconnectivity of flows in the Slavíč catchment in the Moravian-Silesian Beskydy Mountains. ESRI ArcGIS and GRASS GIS tools were used for these analyses. Field verification of outputs took place at several sampling points within the main stream Slavíč.
INTRODUCTION
Together with organic material, sediments form an essential part of fluvial systems and, along with the energy of the flowing water, they shape the varying morphology of the riverbed. Individual parts of riverbed sections or catchments are connected to each other in natural systems, which we collectively call “connectivity”. This research focuses on the geomorphology and material connectivity in torrents, which are usually characterized by high potential energy for water and material transport [1].
Anthropogenic as well as natural structures (e.g. wood jams stabilized by gravel bars) can have a disconnective effect in a watercourse, and thus limit downstream transport of material. However, this does not apply to hydrological connectivity, which is not greatly affected by this disconnectivity. Disruption of hydrological connectivity can be seen to a certain extent in, for example, dam reservoirs where manipulations are carried out, and modified flows enter the watercourse below the reservoir dam, which also has a retroactive effect on sediment connectivity [2].
Unfortunately, a number of negative anthropogenic barriers have been introduced into these naturally functioning systems through human activities, which significantly disrupt these interconnections both longitudinally (retention barrages, stabilization drops, weirs, waterworks, etc.) and laterally (e.g. bank reinforcement). These anthropogenic structures in the catchment cause so-called disconnectivity in different long time scales and with different intensity [2]. Retention barrages, for example, are most effective in the basin immediately after construction and then until the retention space is completely filled with transported sediment. Depending on the intensity of sediment transport, the disconnectivity may only last for a limited period of time, after which material transport may resume. In contrast, for comparison, reservoir dams form an essentially insurmountable and permanent barrier for all sediments that are transported there. This creates a significant problem from the point of view of the downstream connectivity of bedload when, due to the lack of sediment supply to the bed below the dam, together with water management manipulations, it can result in sediment starvation [3].
Research on the connectivity of sediments and material in the catchment can bring us useful information about the erosion-transport-accumulation conditions in the catchment and also help outline appropriate management. In terms of connectivity, source areas of sediments are significant that can be represented by active landslides in the connection of the slopes with the riverbed, and in terms of connection of the banks themselves with the riverbeds they can be represented bank scours. The transport of material takes place along the entire length of the riverbed and is slowed down by natural structures, especially woody matter or local accumulation of sediments. In contrast, sections of the bed with exposed bedrock can act as so-called accelerating zones [2]. Sediment accumulations are formed by fragments of rocks of different degrees of abrasion (according to the length of transport and petrographic composition) and size (gravel, stony to boulder fraction), creating accumulation shapes in the form of so-called gravel bars. Accumulation structures are represented by gravel bars, which, depending on the intensity of flood rate, can be either in a potentially mobile state (with sporadic growth of vegetation) or significantly stabilized by vegetation (trees with a developed root ball). All these erosion, transport, and accumulation processes are most significantly affected by the previously mentioned anthropogenic structures.
Approaches to detecting these erosion-transport-accumulation ratios can be carried out in two steps; the first step involves the identification of potential connectivity using spatially based models [4, 5, 6]; the second step is a field survey, ideally one that is supported by monitoring ongoing processes under different events, especially during floods or droughts.
STUDY AREA
The Slavíč catchment, covering 17.4 km2 (fourth order catchment, ČHP (hydrological sequence number): 2-03-01-0410-0-00) is part of the Oder catchment and extends SE towards the village of Morávka, or E-SE of Morávka water reservoir, at an altitude of about 505 m above sea level. The Slavíč catchment is bordered by the Slavíč, Babí vrch, Kalužný, Smrčina, Ropice, Velký Lipový, and Kyčera hills; these reach an altitude of 834 to 182 m above sea level.
From a geomorphological point of view, the study area is part of the Alpine-Himalayan system, the Western Carpathian province, the Outer Western Carpathian subprovince, the Western Beskydy region, the Moravian-Silesian Beskydy unit, the Lysohorská hornatina subunit, and the Ropická rozsocha district. Lysohorská hornatina covers an area of 362 km2, has an average altitude of 709.9 m, and has an average slope of 14° 45’. It is a rugged rock formation, which is built by the assemblage of Godula and Istebna layers. Traces of periglacial formation represented by boulder chutes, frost cliffs, and pseudokarst fissures can be observed in the relief. Ropická rozsocha is located in the northeastern part of Lysohorská hornatina and represents a rugged mountain range covered with spruce-beech forest [7].
The rock massif is eroded in the axis of the basin, in the E-W direction, by fluvial processes into a deeply incised valley through which the Slavíč stream flows. In the valley floodplain of the watercourse, clayey, sandy, and gravelly fluvial to deluviofluvial sediments are found in the overburden of the rock massif, while in its vicinity there are loam sand to loam stone proluvial and, further from the stream, deluvial sediments of Quaternary age.
Due to the steepness of the slopes, a number of slope deformations in the form of landslides and streams occur in the entire Slavíč catchment, which are both calm and active, i.e., they represent the source area of alluvial material gradually transferred to the Slavíč watercourse.
The Slavíč stream originates on the border of the villages of Morávka and Horní Lomná at an altitude of about 900 m (Fig. 1). It flows through a gravel bed and its length is 7.85 km. It empties from the right side into the Morávka reservoir at an altitude of 505 m above sea level. There is a CHMI gauging station on the watercourse, for which the N-year flows were derived according to ČSN 75 1400 in the range of 5.01–65.8 m3. s-1 (Tab. 1).
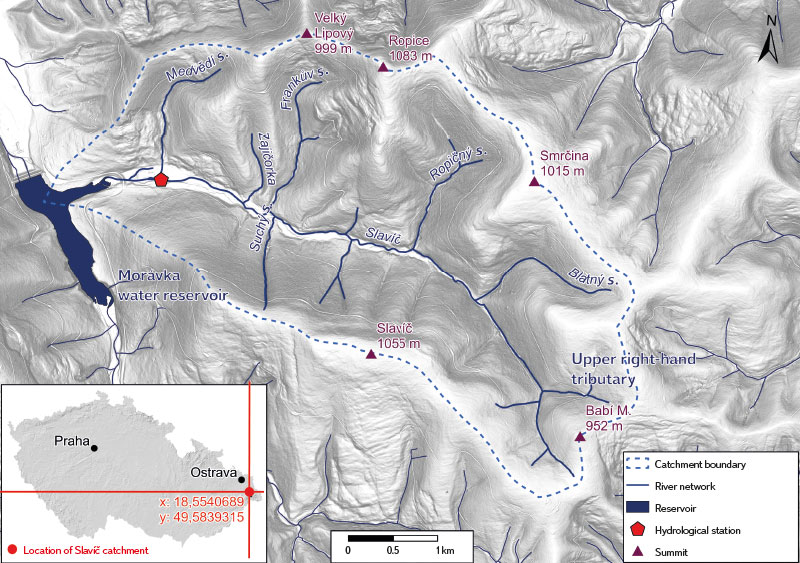
Fig. 1. Study area of the Slavíč river catchment in the Moravian-Silesian Beskydy Mountains
Tab. 1. Slavíč stream discharges with N-year return period (Source: CHMI)
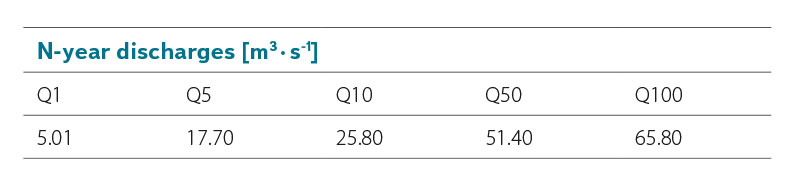
In connection with the main topic of this study, i.e. the modelling of erosion and connectivity of sediments, it is also necessary to mention anthropogenic interventions in the Slavíč catchment, which have a direct influence on its present-day form and the processes in it. In the past, the watercourse was affected by timber rafting and torrent regulation. For the purpose of timber rafting, a splash dam was also built in the upper part of the Slavíč catchment, which served as a control dam, with a length of about 12 m. It was destroyed by a flood in 1880. Other regulatory modifications that also helped timber rafting are dry-stacked stone walls [8]. Practically along its entire length, the stream runs along an asphalt road, so there are culverts, bridges, footbridges, and various bank reinforcements. There are retention barrages and stabilization drops in the watercourse itself. Of the natural elements, there are mainly rock steps, gravel bars, and river wood. Bank erosion and scours can also be observed.
METHODS
Nowadays, there is a number of sophisticated software tools that allow us to model various scenarios based on our chosen requirements and base data. One of these tools for identifying connectivity in the basin is the Connectivity Index Target (CI), which works on the ArcGIS ArcMap platform in the form of a so-called toolbox [9]. The model enables the identification of sediment source areas and the potential connectivity of sediment transport into a channel network. The input data includes a modified, hydrologically correct Digital Relief Model (DMR), a generated river network from the DMR with an envelope layer of an average channel width of 5 m, and a layer of vectorized land use categories (LU), specifically based on an orthophoto from 2016 (ČÚZK – Czech Office for Surveying, Mapping and Cadastre). The result is a map with a rendered colour scale of potential connectivity in a basin, or by connectivity values that are dimensionless. However, depending on coarser resolution, the model cannot identify disconnectivity inside and outside the stream channel (retention barrages, bank reinforcements), which have a smaller area than the individual pixels of the grid. Therefore, when researching connectivity, it is important to know the natural and anthropogenic structures in the basin. Natural and anthropogenic structures located in the channel were identified by a simple method – fluvial-geomorphological mapping of the channel and the surrounding area. To record the occurrence of individual structures, a handheld GPS, type eTrex® 30x, and a measuring tape for measuring the dimensions of individual structures were used.
Verifying connectivity in the context of the whole catchment was also carried out using erosion empirical formulas (USPED), DMR hydrological analyses (Terraflow for GRASS GIS), and dynamic erosion models (SIMWE for GRASS GIS).
The USPED (Unit Stream Power-based Erosion Deposition) method provides us with a detailed insight into the erosion threat in the area – it defines the areas of sediment collection and accumulation in a basin. The input data includes the Digital Relief Model (DMR) layer, Soil Maps 1 : 50 000, and the R factor (Regionalized Erosion Efficiency Factor of Torrential Rain) and is used to calculate the long-term soil loss due to water erosion using the USLE equation (CHMI, VÚMOP). Sheet flow was assumed for the calculation. The calculation itself was performed in the ArcGIS Pro environment using the determined runoff using the Multiple Flow Direction (MFD) method. This method can identify source and sedimentation areas and potential lines of sediment connectivity; however, it is not an erosion model in the true sense of the word in terms of implemented numerical and analytical methods.
The SIMWE (SIMulated Water Erosion) model is part of the GRASS GIS geographic information system. Originally it was only available as an add-on module; since version 6.2.2, it is implicitly included in hydrological analysis tools. The same data enter the model as the above-mentioned erosion model, supplemented with coefficients for surface runoff calculated according to McCuen [10, 15] as a function of vegetation cover and soil hydrologic group and precipitation data. Its output is the spatial distribution of steady sediment flow rate, sediment concentration, and soil erosion/deposition rate. SIMWE is a fully distributed model whose resolution depends on the resolution of the input rasters. The r.sim.water module enables a distributed solution of infiltration and surface runoff. The surface runoff is solved in 2D using the Saint Venant equations and the diffusion wave approximation. The sediment outflow is solved by the r.sim.sediment module [6]. The model produces outputs in raster form.
Base layers for individual analyses are shown in Tab. 2.
Tab. 2. Base layers which were used for individual analyses
Twelve sections of 200 clasts each were measured in the main flow according to Wolman [20] using the granulometric method (Fig. 2). Clasts were sampled randomly from the upper layer from the grain size (D ≥ 2 mm), for which the length of the axis b was always measured. In each section, the channel slope and the geometric parameters (the width and depth of the full channel state) were also measured with an accuracy of 0.1 m. Direct and regularly formed sections of the watercourse (preferably without anthropogenic modifications) were selected for sampling. To determine downstream trends, the percentiles D5, D25, D50, D95 were calculated from the measured values of the b axis lengths (mm) from the entire sample of each section [4]. A percentile is a grain size value that is given by a cumulative distribution curve for a given percentage of “finer particles”. So, for example, “D50 = 30 mm” means that 50 % of the particles in the entire sample have a grain size smaller than 30 mm.
D50 is the median of the distribution curve that divides the sample into two equal parts. The D25 and D75 percentiles are quartiles.
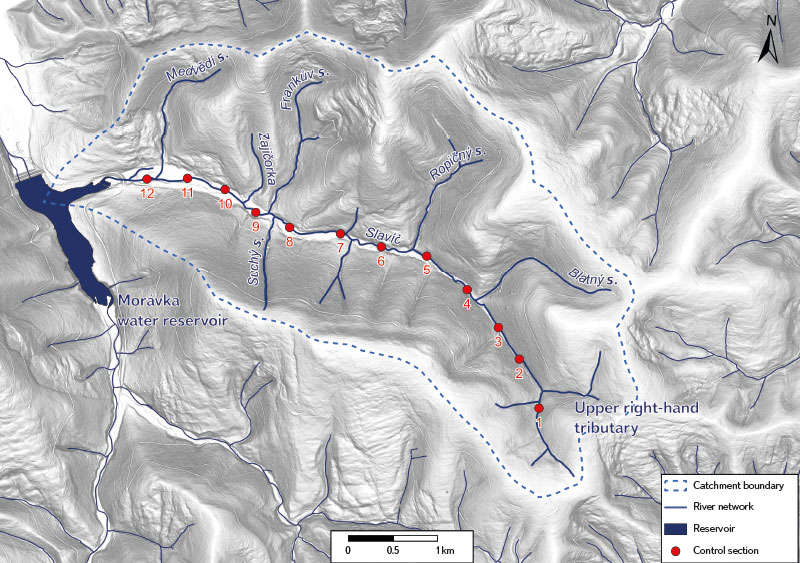
Fig. 2. Sampling points on the Slavíč river using Wolman granulometric measurement method
In addition, in order to determine the potential energy of the riverbed for sediment transport, Stream Power Index (SPI) was calculated. Main factors determining the resulting SPI value include the longitudinal slope of a riverbed (S) and the size of a contributing sub-basin. SPI was calculated to find the potential energy of the stream at the potential flow of Q1. SPI is denoted by the unit ω (Wm−2) and, according to Bagnold [11], bears the following relation:
where:
Q is flow rate (m3 ∙ s−1)
S slope (m/m)
ρ water density (1,000 kg ∙ m−3)
g gravitational acceleration (m ∙ s−2)
W channel width before rated flood (m)
For high-gradient flows, a direct relationship between the SPI during the full channel state (ωbf) and the catchment area (A) with an area of < 10 km2 was demonstrated, when the parameter (ωbf) was simplified to the following form:
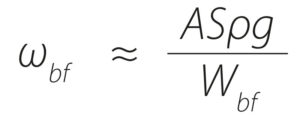
where:
A is the catchment area (km2)
Wbf full channel width,
which reflects the downstream increase in flow rate:
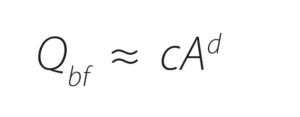
However, in the research conducted by Galia and Škarpich [12, 13], a modified relationship was used for the small basins of the Lichnovský, Lubina, Malý škaredý, and Veřovický streams, which have a similar catchment area and similar morphological, hydrological, and geological parameters as the Slavíč catchment.
Therefore, the same relationship was used in this case:
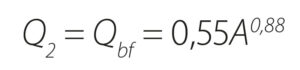
RESULTS
The highest connectivity was manifested near the river network, especially in the tributary areas, where the slopes directly connect to the channel. From the point of view of LU influence, clearcuts were the most significant, which indicate artificially accelerated transport of material into the riverbed. Here, the slopes reach high slope values and the river floodplain has not developed. On the other hand, the lowest connectivity was found in the areas of the individual sub-basins, where the slopes are low and transport of material is probably quite limited. Low connectivity is also characterized by the river floodplain, which forms a natural temporary barrier for the transport of sediments into the channel. In the case of the main watercourse, the south-facing slopes are better connected to the bed because the left bank of the watercourse has a wider floodplain than the right bank. The resulting connectivity map is shown in Fig. 4.
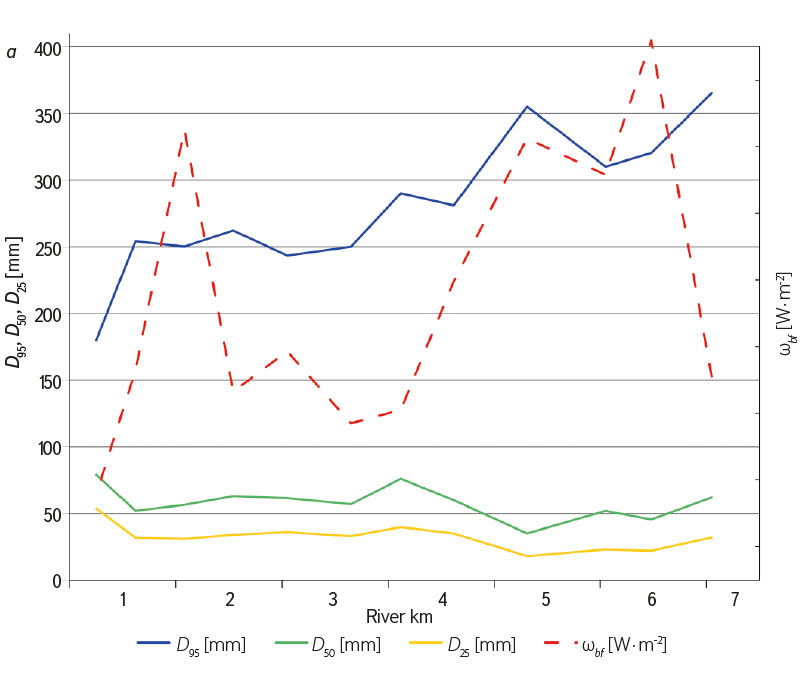
Fig. 3. a) Downstream grain size trends of sediments D25, D50, D95 and SPI;
b) downstream trends of width (Wbf ) and slope (S) of the Slavíč channel
Fig. 4. Output connectivity map of the Slavíč catchment, which are combined methods of Connectivity Index Target, identified clearcuts, and terrain mapping
Fluvial-geomorphological mapping revealed a large number of natural and anthropogenic structures in the channel network of the Slavíč catchment. The mapping results are presented in Tab. 3.
Tab. 3. Results of fluvial-geomorphologic mapping in the Slavíč channel network
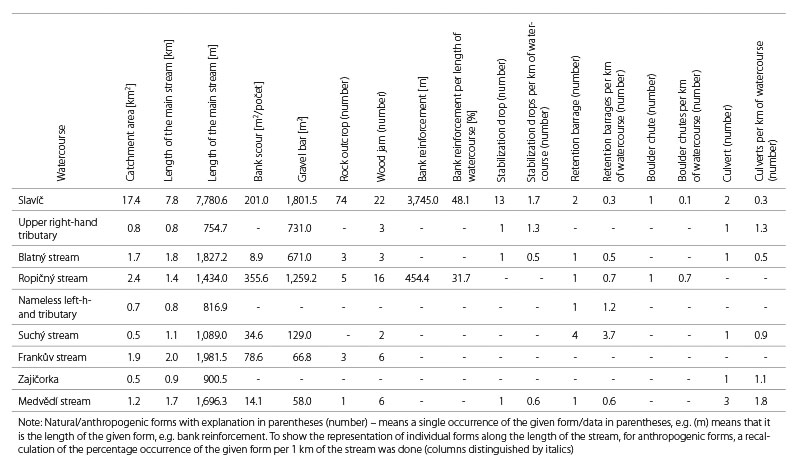
The results of the granulometric method showed downstream refinement of clasts with a grain size of D95 with a sudden increase in the fourth section. On the other hand, the D5 percentile values increase downstream with a slight fluctuation, and a sudden drop was also manifested in the fourth section. SPI generally shows higher values in the upper part of the channel, however, depending on the slope, these values fluctuate considerably. A sudden increase was evident in the second and tenth sections (Fig. 3).
When compared to the outputs of erosion models, it is possible to see that the modelling of mountain basins gives very similar results even when using different methodologies (static vs. dynamic models), which correlate with the results of sediment connectivity modelling. All three types of models used can reliably model potential sites of sediment concentration and contributing areas.
Erosion modelling by USPED and SIMWE shows the sensitivity of the mountain (mainly forested) catchment model especially in open terrain. Due to the characteristics of the terrain relief with large slopes and their local changes, the effects of other factors (land cover, soil, and erosion efficiency of rain) are erased. The output of the USPED method for the Slavíč catchment is shown in Fig. 5. The result of the SIMWE model in the form of soil erosion/deposition for the study area is shown in Fig. 6.
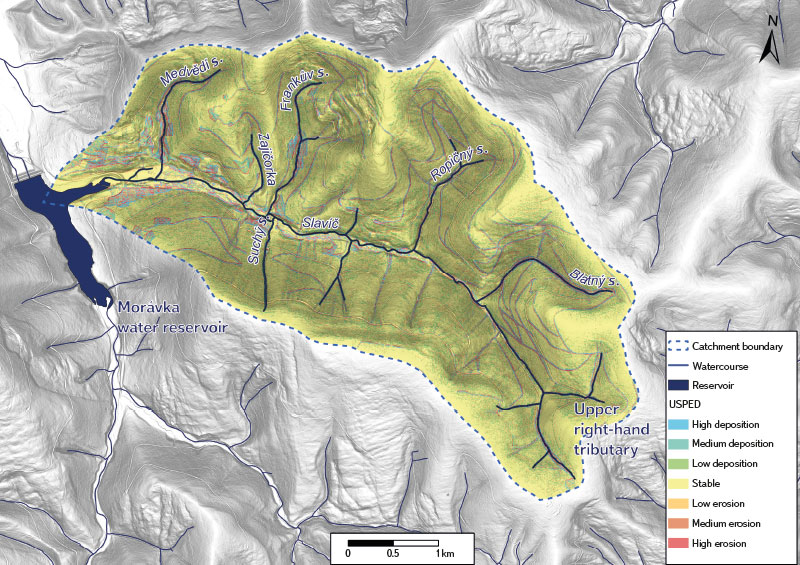
Fig. 5. Resulting map of the erosion and accumulation rate using the USPED method within the Slavíč catchment
Fig. 6. Model output of SIMWE for GRASS GIS catchment presented in ArcGIS Pro representing erosion and deposition rates within the Slavíč river catchment
The combination of Connectivity Index Target, USPED and SIMWE models, supplemented by fluvial-geomorphological mapping of the riverbed and the surrounding area, helps us connect the methods of identifying erosion areas as well as hydrological connectivity with sediment transport connectivity, which is strongly linked to longitudinal and lateral disconnective structures in the riverbed. All the models used are a static image of the real situation in the basin and are dependent on the details and accuracy of the input data. The Connectivity Index Target model only deals with connectivity and sediment movement routes in the basin; the USPED and SIMWE models also work with hydrological connectivity. The advantages of the individual models lie in the fact that they are not demanding on the amount of input data and relatively fast calculation. For comparison, in the outputs of the Connectivity Index Target and SIMWE models, the potential routes of sediment transport are clearly visible, and both are also able to identify the deposition zones, which are shown in blue in both models. Moreover, the SIMWE model can recognize out-of-channel erosion, which is shown in red. The resulting USPED map differs from the other two models in that it has a clearly identifiable influence of forest roads, which act as erosion paths for sediment transport.
DISCUSSION
In this study, modelling approaches were used in combination with fluvial-geomorphological mapping and the granulometric method. Each of these approaches has its advantages and disadvantages. Modelling software gives us a quick insight into potential connectivity in a basin; however, for proper interpretation, it needs to be complemented by other methods, in this case field survey. The field survey revealed a lot of out-of-channel disconnectivity affecting sediment transport. This method brings very detailed results when done correctly, but it is quite time-consuming.
Last but not least, it is important to obtain data on the grain size of the sediments, from which, in combination with the previously mentioned methods, it is possible to interpret, for example, the effect of disconnectivity on the downstream connectivity of sediments, to reveal sediment starvation, etc. The disadvantage of granulometric methods also lies in the time-consuming nature of not only analysing clasts in the field, but also in the subsequent statistical processing of the measured values.
By modelling connectivity using the Connectivity Index Target (CI) tool, it was proven that the slopes are quite well connected with the riverbeds in terms of sediment transport. High connectivity values in the tributary area are mainly due to high values of slope gradients, in many places supported by anthropogenic LU change. Clear-cutting has a negative effect on the stability of the slopes, which are easily eroded, and thus a large amount of wood material, sediments and fine soil particles are transported into the watercourse, where, especially during the period of increased rainfall, gully erosion occurs and the soil is flushed into the riverbed.
The river floodplain located especially in the lower part of the main stream acts as a natural buffer zone, which due to its low slopes limits the transport of material from the slopes to the channel [2]. However, a major problem in the context of the entire Slavíč catchment is the occurrence of the Morávka reservoir, where all material transported from the higher parts of the basin ends its route.
In the Slavíč catchment, field mapping revealed a large number of disconnectivities, mainly of anthropogenic origin. The most significant disconnective elements include retention barrages located especially on tributaries (Fig. 4). In certain cases, e.g. in the Suchý stream, there is a large number of old barrages whose retention space is already largely filled up, and the original retention function is thus significantly limited. As for the supply of sediments to the riverbed, the most important structures are bank scours, which are mostly situated on tributaries. These scours are of an active nature and thus supply the riverbed with material in the case of increased flows. Accelerating zones occur most often in the main bed in the form of rock outcrops and thus make transport more efficient. The numerous occurrence of these rock outcrops can be attributed to the cumulative effect of anthropogenic structures, especially bank reinforcements, which prevents the supply of material from the banks to the riverbed, and thus the gradual washing out of sediments and their downstream coarsening occurs. Stabilization drops also have an effect on the coarsening of sediments, which was found in the research conducted by Galia et al. [12]. However, stabilization drops do not always mean slowing down of sediment transport; by canalization and channel modifications, the transport can be accelerated, which was found during research on the Malý Lipový and Bystrý streams [13, 14]. Last but not least, the accumulation zones are represented by gravel bars, which are dynamic and during the flood season they regularly overflow and transport material further down the stream.
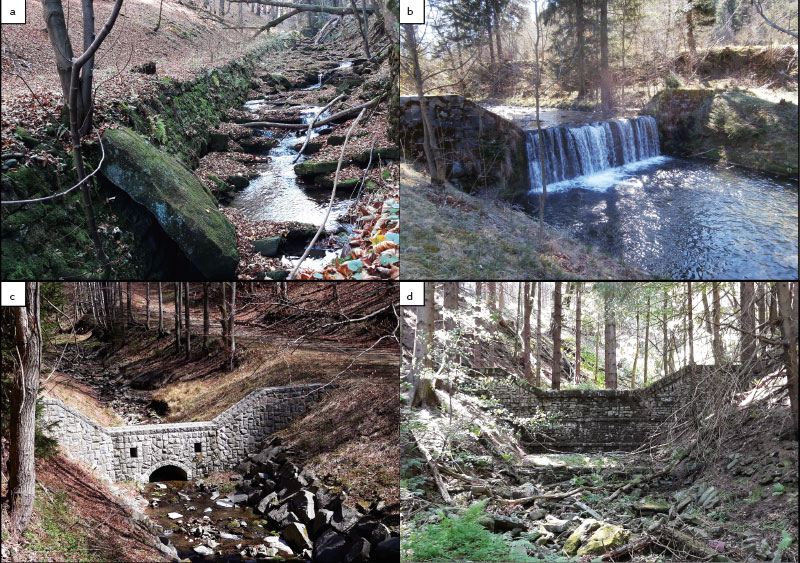
Fig. 7. Anthropogenic disconnectivity within the Slavíč catchment: a) old embankment on the upper part of the Slavíč stream; b) stabilization step on the lower part of the Slavíč stream; c) retention barrier build on the Ropičný stream; d) old retention barrier on the source area of the Slavíč stream
A natural feature of torrents is the episodic transport of material, especially during floods, and therefore it is important to look at the transport of bed-load from a long-term perspective, e.g. in the context of several consecutive flood events during the last decades. The main stream bed has a high potential energy for sediment transport in the upper part. Unfortunately, this is limited by low flows under normal hydrological conditions or during dry season. In such a period, the bed can be described as a channel with a limited transport capacity [17]; there is a sufficient amount of material, but no energy to transport it.
The SIMWE model is a relatively powerful model; its main advantages include multi-scale simulation, which, within the small resolution of the study area, allows for a more detailed solution of a certain part of the area using dynamic sampling points, so-called walkers. Another advantage is the modification of the wave approximation equations for higher stability of the solution in DMR areas with a lower slope and hydraulic gradient or in areas with a difficult to determine flow direction, e.g. in a terrain depression.
The SIMWE model for GRASS GIS represents a suitable tool for the evaluation of rainfall-runoff relations together with erosion and transport-accumulation processes. However, it is necessary to verify its outputs; the version for Linux OS shows better calculation stability than the version for OS Windows, which can be reflected in the functionality of the program itself and sometimes also in the actual numerical values of the results. For more detailed analyses, it will also be appropriate to use the simulation of sediment transport in the hydraulic models HEC-RAS and MIKE 11, while the latest version of the HEC-RAS model offers increasingly proficient tools in this regard.
CONCLUSION
In conclusion, it can be stated that, among other things, by combining both methods (i.e. spatially based modelling of potential connectivity using GIS software and terrain mapping) it is possible to deal with the connectivity of sediments in mountain basins effectively. However, the research verified the importance of fluvial-geomorphological mapping, which brought detailed results about the occurrence of natural and anthropogenic structures in the riverbed and their influence on the morphology and downstream trends of sediments; generalized input geographic data and model results showing potential connectivity of sediments do not give a comprehensive picture of the real state of the basin and the watercourse itself. The Slavíč catchment is a significantly anthropogenically modified basin, especially in the area of the main stream. However, some tributaries (e.g. Frankův stream) have very little anthropogenic modification, and there is a semi-natural character of the bed with a lot of organic material. The tributaries with their numerous bank scours serve as the main sediment source zones. Connectivity modelling together with the results of field mapping provided an insight into erosion-transport-accumulation conditions in the basin and, simultaneously, showed the degree of anthropogenic influence. Analyses on other pilot catchments (Slučí, Sokolí, Suchý stream in the Černá Opava catchment, and Svinný stream in the Osoblahy catchment) will undoubtedly bring interesting data for comparison, among other things with regard to the different lithological and geomorphological conditions of the catchment.
Acknowledgements
The authors would like to thank the projects NAZV QK22010189 DEFOREST “Impact of deforestation on the water regime of small catchments” and NAZV QL24010054 “Impacts of climate change on small forest catchments and the possibilities of their mitigation through forestry management and water management measures”; without their support, writing the article would have taken much longer and in a more complicated manner. They would also like to thank to DKRVO (Long-term concept for the development of a research organization for 2023–2027) CHMI, especially to areas 6 and 12.
This article was translated on basis of Czech peer-reviewed original by Environmental Translation Ltd.