The increase in potential evapotranspiration due to warming is quite often used as an indicator of ongoing and predicted changes in the hydrological balance. However, without assessing its effect in basins with different precipitation regimes, it is not correct to consider a change in potential evapotranspiration as an increase in actual evapotranspiration or a decrease in runoff.
For a better understanding, let us repeat the definitions of the basic components of the hydrological balance which we will use in the following considerations and calculations:
- PRECIPITATION in the form of rain, snow, hail, dew, hoarfrost, frost, etc. is the only input of the hydrological balance.
- EVAPOTRANSPIRATION includes evaporation (evaporation from water surface, soil, and wet plant surface) and plant transpiration.
- POTENTIAL EVAPOTRANSPIRATION is the theoretical upper limit of evaporation from a surface under given natural and meteorological conditions. It expresses the ability of the air environment to remove water from the surface – it corresponds approximately to evaporation from a free water surface or evapotranspiration from grassland with optimal humidity. It depends mainly on the air temperature.
- ACTUAL EVAPOTRANSPIRATION depends on air temperature, or potential evapotranspiration, and is limited by the amount of water available for evaporation and transpiration.
- SURFACE RUNOFF occurs in two situations – if the intensity of precipitation exceeds the rate of infiltration, or if the upper soil profile is completely saturated with water. It reaches the nearest watercourse quickly, within minutes to hours.
- HYPODERMIC (SUB-SURFACE) RUNOFF takes place through preferential paths in the upper layer of soil and subsoil without contact with the groundwater level. It enters the watercourse within a few days after rain or snowmelt.
- BASEFLOW (UNDERGROUND RUNOFF) is water flowing from groundwater storage in the form of springs or hidden under the surface into watercourses and reservoirs. It manifests itself in a watercourse within weeks to months after rain or snowmelt.
- WATER STORAGE is found in groundwater collectors, soil, wetlands, water reservoirs, and snow and ice, usually for a temporary period. If we deal with averages from a sufficiently long multi-year period, the influence of water storage can be omitted; however, it is significant when assessing the balance of individual years or shorter periods.
The hydrological balance of the basin, which can be expressed by the following equation, includes actual, not potential, evapotranspiration:
PRECIPITATION = EVAPOTRANSPIRATION + SURFACE RUNOFF + SUB-SURFACE RUNOFF + UNDERGROUND RUNOFF ± CHANGE IN WATER STORAGE (Fig. 1)
The hydrological balance of a basin can be described as a competition between two output components – evaporation from the basin surface and water runoff from the basin for a share of the input component, which is atmospheric precipitation. Here, ‚runoff‘ means the total runoff, created in time-varying proportions by the component of surface runoff, sub-surface runoff, and groundwater runoff/baseflow, concentrated in the flow through a closing profile of a watercourse.
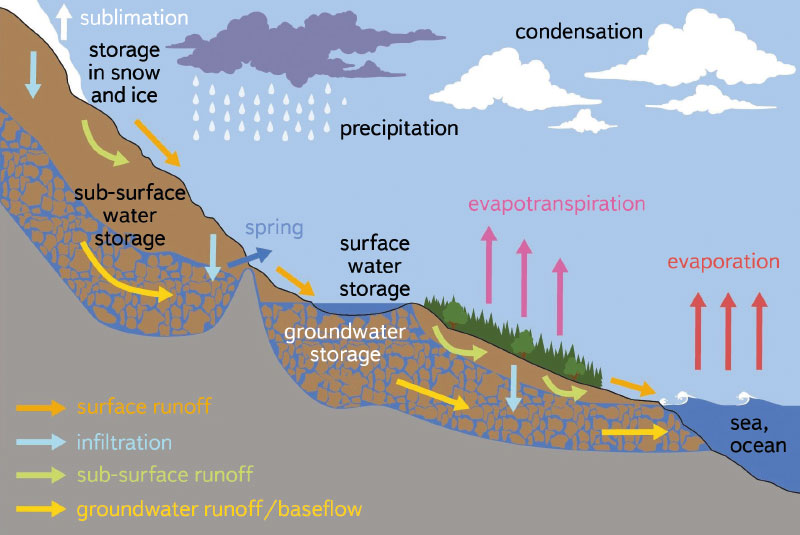
Fig. 1. The hydrological cycle [2]
The long-term average annual precipitation in the Czech Republic is 680 mm, actual evapotranspiration is 490 mm, total runoff 190 mm; i.e., only 28 % of precipitation, while 72 % of precipitation evapotranspirates. In our climatic conditions (with the exception of surface runoff from torrential rain, which on a long-term average makes up about 2 % to 6 % of precipitation) the decisive part of the balance is influenced by actual evapotranspiration, which depends on potential evapotranspiration, but is limited by the amount of precipitation and the available amount of water in the soil surface layer, which is the result of the balance of the previous period.
Potential evapotranspiration
There are several calculation methods for determining potential evapotranspiration. In the field of hydrological modelling, a relatively simple procedure according to article [1] has proven itself, in which its course is determined by air temperature. Potential evapotranspiration is given in the same units as precipitation, i.e., the amount of water that has fallen or evaporated per year or month. Due to its dependence on air temperature, potential evapotranspiration has a typical course during a year, shown in Fig. 2. It shows the long-term average monthly amounts of potential evapotranspiration during the hydrological year in the Labe basin in Děčín. As an example of its variability in individual years, values are shown for the extremely cold year 1941 and the very warm year 2015. The biggest difference between the values from 2015 and 1941 is in August – it is 26.5 mm and corresponds to 30.8 % of the value in 1941. Greater differences in potential evapotranspiration are shown in Fig. 3, which shows its course in the Rakovnický stream basin in central Bohemia in the extremely warm year 2018 and the course in the Chomutovka mountain basin below Třetí Mlýn in the relatively cold year 1987. The biggest difference in the courses is in July: 36.9 mm, i.e., 38.5 % of the value in Rakovník.
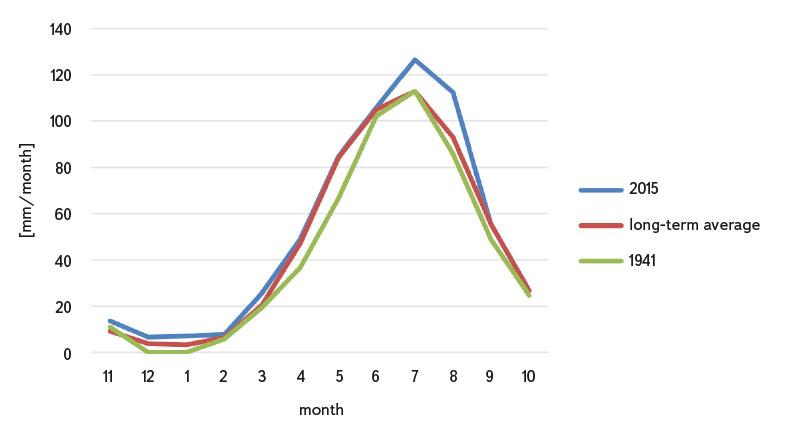
Fig. 2. Course of potential evapotranspiration in the Labe basin below Děčín in the warm year 2015, in the extremely cold year 1941, and in the long-term average
Fig. 3. Course of potential evapotranspiration (PET) in the warm year 2018 in the Rakovnický stream basin and in the cold year 1987 in Chomutovka basin
Actual evapotranspiration
Examining regression relationships for estimating annual evapotranspiration levels showed that for most basins, the relationship between evapotranspiration and precipitation is significantly tighter than the relationship between evapotranspiration and potential evapotranspiration. In the regional estimation of long-term averages of actual evapotranspiration (i.e., also long-term runoff averages), relations using precipitation and temperature as explanatory variables are usually applied. The relationship between potential evapotranspiration and precipitation over time is a decisive factor for the amount of actual evapotranspiration, the replenishment of water in the soil, and the formation of runoff. To describe the hydrological balance, we choose a monthly step, in which the balance storage components compensate for the more detailed fluctuations of the balance quantities. During the balance process, two different situations occur:
When the distribution of precipitation in a month is omitted, the assumption can be used that if precipitation in a given month exceeds potential evapotranspiration, part of it is consumed for actual evapotranspiration equal to potential evapotranspiration, and the remaining part infiltrates into the soil. If the soil is fully saturated, some precipitation will percolate through the soil, generating sub-surface runoff and replenishing groundwater storage.
If precipitation in a given month is less than potential evapotranspiration and the soil is saturated, all of it is used for actual evaporation. If the soil is not completely saturated, some precipitation will increase the water storage in the soil, some will contribute to evaporation, but actual evaporation is less than potential evapotranspiration.
Considering the consequences of ongoing warming, it should not be forgotten that an increase in air temperature, or an increase in potential evapotranspiration, is manifested by an increase in actual evapotranspiration only when water from precipitation and from the soil storage is available for evaporation and transpiration. Such conditions will more probably occur in basins at higher altitudes where precipitation is greater. As a result of lower temperatures, there is less potential evapotranspiration in basins at higher altitudes, so the described condition is more easily met.
Examples of courses of balance quantities in relation to potential evapotranspiration
Monthly values of precipitation, potential evapotranspiration, evapotranspiration, and runoff in the cold year 1986 and the warm year 2015 are shown in Figs. 4 and 5 from the Chomutovka mountain basin (43.6 km2), and in Figs. 6 and 7 from the Rakovnický stream basin (302 km2). Tabs. 1 and 2 contain the annual hydrological characteristics of listed years. Annual evapotranspiration values correspond to monthly modelled courses shown and differ from the differences (precipitation minus runoff) by empting or filling water storage in the basin (the largest decrease of 46.7 mm in 2015 in the Rakovnický stream basin). Tab. 3 shows an analogous data set for the Labe basin in Děčín, presented without the corresponding figures.
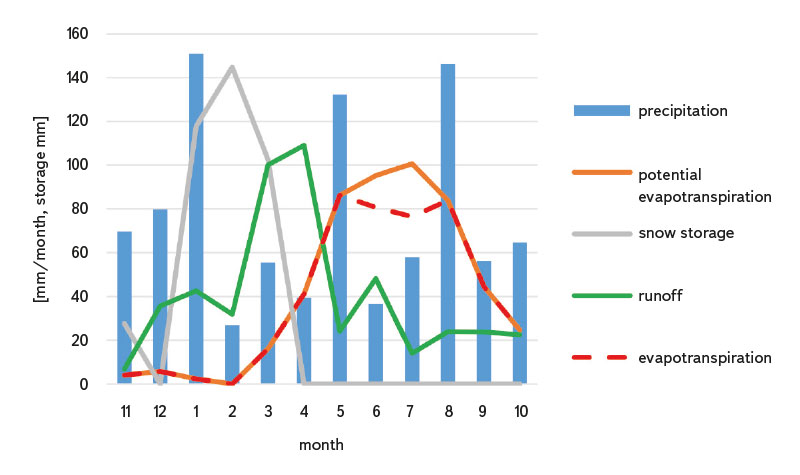
Fig. 4. Course of balance hydrological quantities in 1986 – Chomutovka basin
Fig. 5. Course of balance hydrological quantities in 2015 – Chomutovka basin
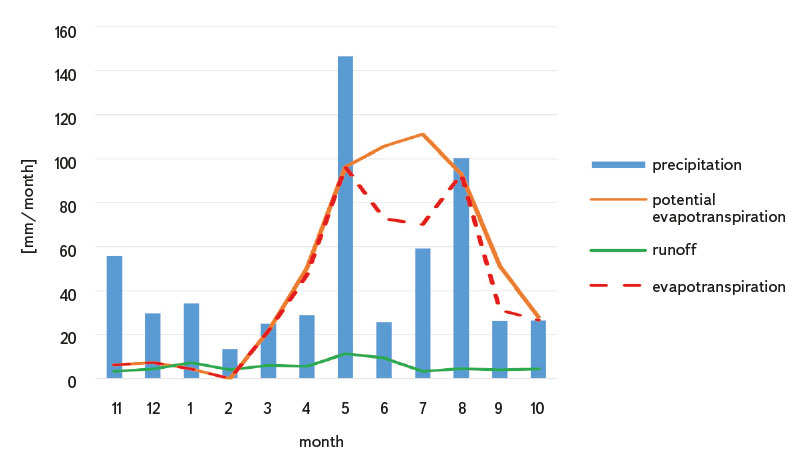
Fig. 6. Course of balance hydrological quantities in 1986 – Rakovnický stream basin
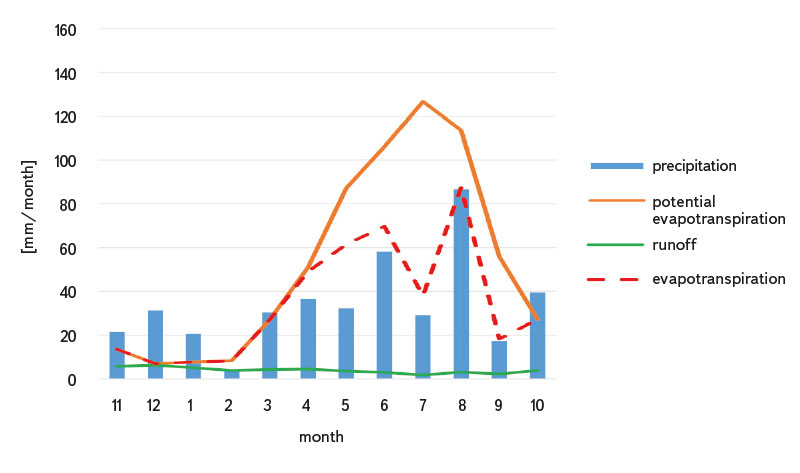
Fig. 7. Course of balance hydrological quantities in 2015 – Rakovnický stream basin
Tab. 1. Characteristics of the hydrological years 1986 and 2015 – Chomutovka basin below Třetí Mlýn
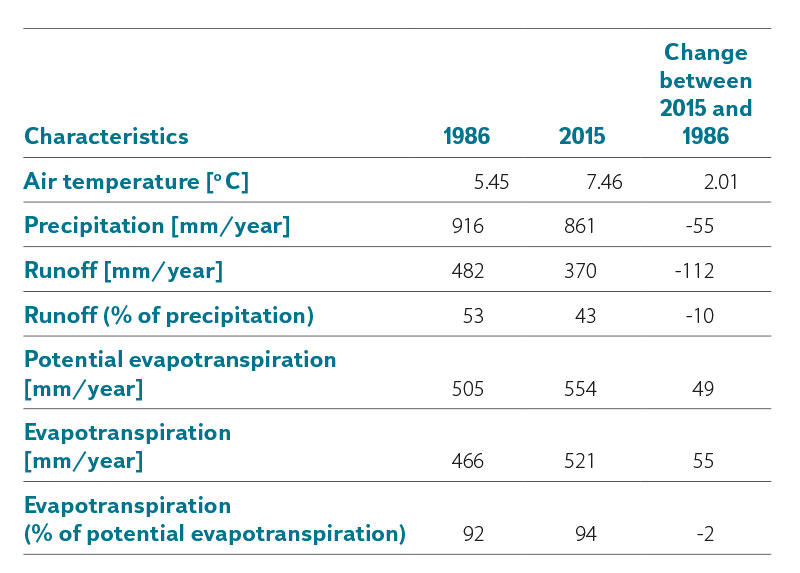
Tab. 2. Characteristics of the hydrological years 1986 and 2015 – Rakovnický stream basin below Rakovník
Tab. 3. Characteristics of the hydrological years 1986 and 2015 – Labe basin below Děčín
In the Chomutovka basin in the cold 1986, potential evapotranspiration exceeded precipitation in only two months, so evapotranspiration was only 8 % less; even in the warm 2015, it was only 6 % less. The increase in evapotranspiration in 2015 compared to 1986 is similar to the increase in potential evapotranspiration. The decrease in runoff is greater, it is contributed by the decrease in precipitation and evapotranspiration.
In the Rakovnický stream basin, even in the cold 1986 (especially in June and July), potential evapotranspiration significantly exceeded precipitation; evapotranspiration was 17 % less. In the warm and dry 2015, it was 34 % less. The increase in potential evapotranspiration in 2015 compared to 1986 did not materialize; as a result of the decrease in precipitation by 148 mm, actual evapotranspiration decreased. The decrease in runoff is small compared to the increase in potential evapotranspiration, even in view of the decrease in precipitation. Evidently, the runoff, approaching the drying up of the stream, was formed by the outflow of the rest of the dynamic groundwater storage and was supplemented by precipitation only until January. It can be seen in Fig. 7 that, even if in 2015 the precipitation from May to July had been significantly greater, it would not have resulted in a significant increase in runoff: it would have been consumed by evapotranspiration.
In the Labe basin, even in the cold 1986, evapotranspiration was 9.4 % less than potential evapotranspiration, and in 2015 33.1 % less, so the limitation of evapotranspiration by the amount of precipitation is manifested here. A decrease in precipitation and an increase in potential evapotranspiration contribute to the decrease in runoff.
The given examples of the course of the hydrological balance in basins with different precipitation regimes show that data on the increase in potential evapotranspiration can, without assessing the effect of precipitation, even if it does not change, characterize the effect of warming only in basins with relatively high precipitation. In most of the Czech Republic, the long-term average of potential evapotranspiration is greater than the long-term average of precipitation, especially in the summer half-year (Fig. 8). In this predominant part of the country, the change in actual evapotranspiration, or runoff, cannot be equated with the change in potential evapotranspiration without further analysis of the hydrological balance.
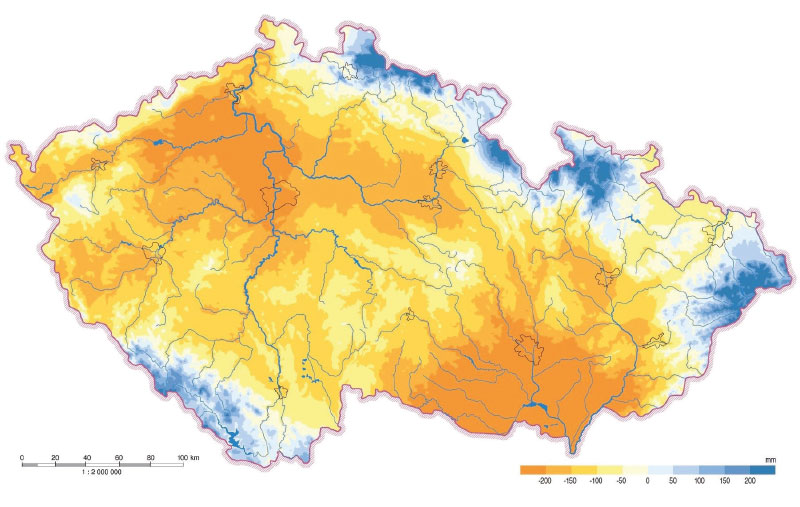
Fig. 8. The difference between total precipitation and potential evapotranspiration in the summer half-year (May to October) in the Czech Republic according to [3]
Consequences of differences in the hydrological balance in the summer and winter half-year for the decrease in flows due to warming
Throughout the Czech Republic, there are significantly different relationships between precipitation and potential evapotranspiration in the cold part of the year. In the winter half-year, precipitation exceeds potential evapotranspiration, so that at positive air temperatures, the soil profile becomes saturated during the winter, and at negative temperatures, snow storage is created. Groundwater storage is supplemented in both types of winter regime. It is more favourable for the further temporal development of runoff when most of the water percolate through the soil later. However, with rapid snowmelt, groundwater storage may be depleted by surface and intensive sub-surface runoff.
To assess the differences in the hydrological balance in the winter half-year (November to April) and in the summer half-year (May to October), we used data from the Labe basin in Děčín. Tab. 4 shows the long-term average values of balance characteristic.
Tab. 4. Long-term averages of precipitation and runoff for the Labe basin in Děčín for the winter and summer half-years, quantities except ratios are in units [mm/half-year]
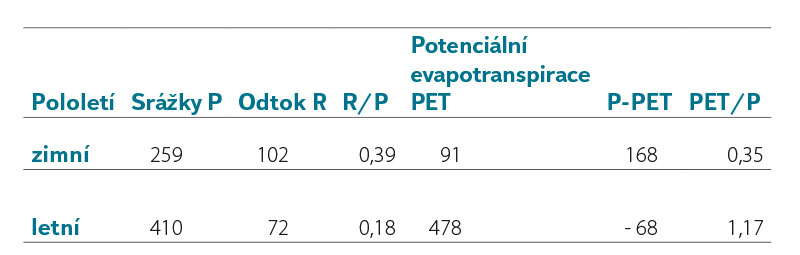
In the winter half-year, precipitation accounts for 63 % of summer precipitation, but potential evapotranspiration is only 19 % of the summer value. Precipitation outweighs potential evapotranspiration. The winter runoff is 1.42 times greater than the summer runoff.
From the point of view of the predicted further warming, it is important that not only the magnitude of potential evapotranspiration, but also the gradient of its increase due to the increase in air temperature is significantly smaller in the winter months than in the months of the growing season (Figs. 9 and 10). The gradients shown on them were derived using the method of calculating potential evapotranspiration according to [1]. Not only from the results of hydrological observations, but also from the theoretical justification, it is clear that summer runoff will decrease significantly more than winter runoff with continued warming.
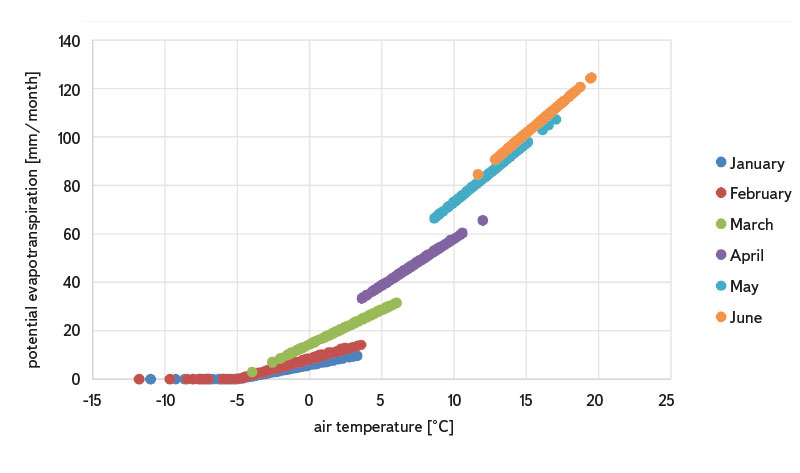
Fig. 9. Relationship between temperature and potential evapotranspiration in individual months of the first half-year
Fig. 10. Relationship between temperature and potential evapotranspiration in individual months of the second half-year
To estimate the consequences of warming, we assessed how the balance quantities changed in the Labe basin in 1991–2019 compared to the corresponding values from the period 1961–1980. 1980 is close to the beginning of an intensive rise in temperatures during the long-term fluctuation of air temperatures. Tab. 5. shows the averages of balance quantities from both periods and their differences (data from 1991 to 2019 minus data from 1961 to 1980). Precipitation increased very slightly in both half-years. In the winter half-year, a very small increase in precipitation outweighed the effect of increased potential evapotranspiration, and even with a warming of 1.1 °C, runoff increased slightly.
Tab. 5. Characteristics of the hydrological balance of the Labe basin in Děčín – winter and summer half-years
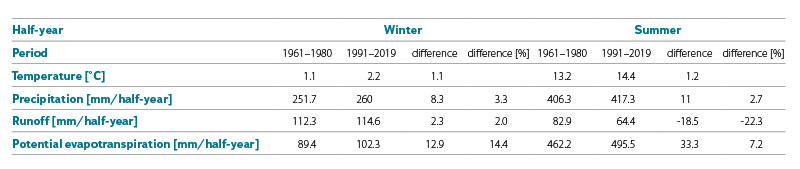
A rise in potential evapotranspiration by 33 mm in the summer half-year with an increase in temperature of 1.2 °C resulted in a decrease in runoff by 18.5 mm, i.e., by 22.3 %. When we estimate the effect corresponding to an increase in precipitation of 11 mm according to a runoff coefficient of 0.18 × 11 = 2.0 mm, we get an estimate of the runoff decrease due to warming of 18.5 + 2.0 = 20.5 mm/year
at a temperature increase of 1.2 °C. When reducing to a change in temperature by 1 °C, we estimate the gradient of the decrease in the average runoff in the summer half-year is 17 mm when warming by 1 °C.
It is clear from the above results that the risk of a decrease in flows due to warming is significantly greater in the summer half-year, when, in addition, temperatures rise more than in the annual average. With continued warming, runoff in the summer half-year will decrease to an ever greater extent, the hydrological drought will be prolonged and deepened, and it will be necessary to use more water transferred from the winter half-year in the summer half-year for water abstractions and the preservation of ecological flows. This cannot be ensured other than by using storage reservoirs. It is therefore necessary to assess to what extent it will be sufficient to ensure this function of the existing reservoirs at the expected intensity of warming. Due to the long time required to build reservoirs (from the plan to the actual construction), this task must be addressed well in advance.
Assuming a temperature rise of 1 °C over 30 years and a uniform decrease in runoff with the indicated gradient of 17 mm/1 °C, the average long-term summer runoff from the Labe basin in Děčín in 2060 would be around 30 mm. For comparison, the minimum average summer runoff so far observed in a single year (2018) was 22 mm in 169 years.
This article was translated on basis of Czech peer-reviewed original by Environmental Translation Ltd.