Summary
This study presents interim results of an evaluation of a potential climate change impact on the preservation of drinking water demand provided by water reservoirs in the timeframe of the year 2050. Hydrological and water sources and demands balance procedures have been applied, including modelling of the storage ability of water resources and water supply systems. A potential climate change impact on hydrological characteristics and subsequently on the security of water demand has been evaluated in a total of 45 water reservoirs in the Czech Republic. A risk of insufficient supply of current drinking water abstraction requirements in climate change conditions has been identified in 17 of the evaluated water reservoirs.
Introduction
Drought together with floods belong among extreme hydrological phenomena that occur naturally and randomly. However, with increasing climate change impacts, frequency of the occurrence, temporal and spatial distribution of extreme hydrological phenomena may change. The results of modelling climate change impacts for the Czech Republic predict a more frequent occurrence of flash floods and long-lasting drought. In recent years, this fact has been proven in many catchment areas. An unfavourable situation may even lead to putting the reliability of drinking water supply for citizens at risk. A significant part of drinking water demand in the Czech Republic is provided by water reservoirs. According to current data recorded for the sake of water balance compilation [1], approximately 50% of the total water demand for public water mains (92% of surface water for public water mains) are provided for by water reservoirs (both by water abstraction directly from water reservoirs and by ameliorating discharge to water abstraction sites at lower altitudes).
The presented evaluation of the preservation of drinking water demand provided for by water reservoirs has been made within project No. VI20192022159 “Water Resources and Water Supply Systems and Preventive Measures to Reduce Risks for Drinking Water Supply” under a Ministry of the Interior programme No. BV III/1-VS. The T. G. Masaryk Water Research Institute is the project investigator. Work on the project was launched in July 2019 and is envisaged to be completed in December 2022. The project focuses on evaluating risks of drinking water supply as a result of climate change and on devising technical tools for assessing possible measures to mitigate potential unfavourable impacts.
Methodology and Material
The assessment of potential climate change impacts on the preservation of drinking water demand from water reservoirs is based on a methodology [2]. Procedures for processing hydrological and water management balance stated in the methodology have been applied in the project, including simulation modelling of the storage ability of water management systems (water reservoirs). The assessment has been made for the prospective period of 2050.
The climate change impact on hydrological characteristics and on the security of water demand have been assessed in water reservoirs that are recorded as water supply ones pursuant to [3] (with an exception of water reservoirs Husinec, Jezeří, Boskovice and Fryšták, which are at present not used for drinking water abstraction). At the same time, the security of drinking water demand from water reservoirs Seč, Vranov and Slezská Harta has also been assessed. Besides water abstraction from these reservoirs, drinking water demand has also been assessed on watercourses where discharge from water reservoirs is actively ameliorated for the sake of securing water demand (the Plzeň water treatment plant on the Úhlava River from the Nýrsko water reservoir and the Milíkov water treatment plant on the Mže River from the Lučina water reservoir) or transferred (the Šumná water reservoir on Bílý Brook from the Fláje water reservoir). Water transfers from Třebízský Brook and from the Podhora water reservoir to the Mariánské Lázně water reservoir have been considered, from Černá voda Brook to the Přísečnice water reservoir and from the Fláje water reservoir to Pekelský Brook (for the Šumná water treatment plant), from Lužec Brook to the Jirkov water reservoir and from Jiřínský Brook and Jedlovský Brook to the Hubenov water reservoir. When the storage function was simulated, the possibility of cooperation or mutual replaceability of water reservoirs Karhov – Zhejral, Podhora – Mariánské Lázně, Vranov – Znojmo, Seč – Křižanovice, Slezská Harta – Kružberk and Morávka – Šance has also been considered.
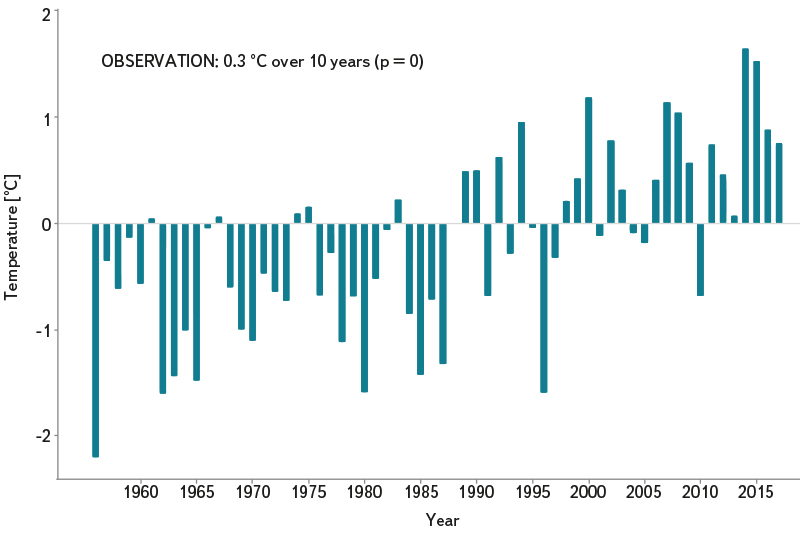
Fig. 1. Mean annual air temperature during the period of 1955–2017 (a statistically significant trend of 0.3 °C over 10 years)
Current Climate Conditions
Data from the period of 1941–2017 have been used for the actual evaluation of current conditions, namely time series of air temperature, precipitation totals and runoff. Fig. 1 presents deviations of mean annual air temperature from the mean annual temperature over the reference period of 1981–2010 (represented by a yellow polygon). A significant temperature increase can be observed, especially in recent years. This temperature increase is statistically significant with a high degree of significance. The green colour represents periods of 3 most significant droughts in this period. Increasing temperature has an impact on the amount of potential evapotranspiration and if water is available in the soil profile, then naturally also on the actual evaporation.
By analogy, the following figures present precipitation totals and runoff heights. Fig. 2 shows the differences of precipitation totals from the reference period. We can see that the periods when precipitation totals were lower (1969–1974, 1989–1994, 2014–2017) repeat themselves and that the deficit was even higher than in the past five years. However, an evaluation of annual precipitation totals does not yield a trend that would be statistically significant. This fact may also be derived from Fig. 3, which presents runoff heights. From the long-term perspective, no statistically significant trend can be established in mean annual runoff (that capture mean values for the whole territory of the Czech Republic). Nevertheless, a significant decline in runoff in the summer and spring months and an increase in January may be observed (which is in particular due to an increase in temperature – liquid precipitation and snow melting). For illustration, a change of runoff by 1 mm means a reduction by 78.87 million m3. This value corresponds nearly to the water volume in the Rozkoš water reservoir.
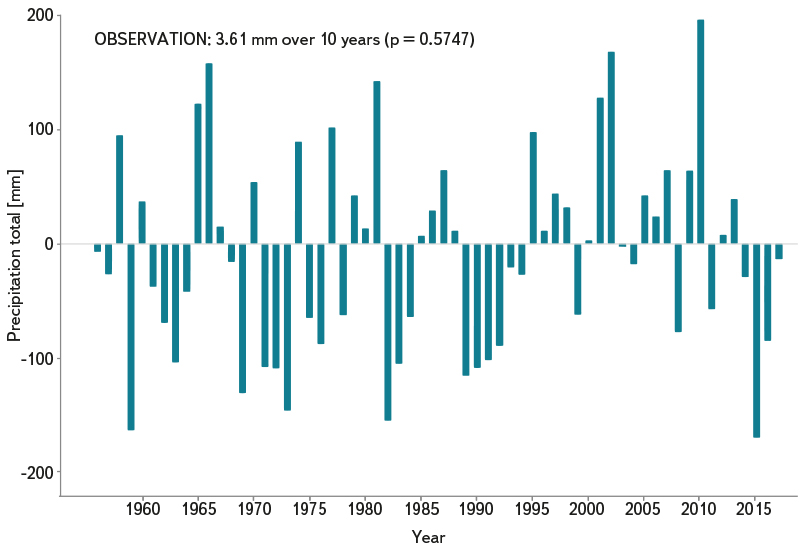
Fig. 2. Mean annual differences in precipitation totals (from normal) for the period of 1955–2017 (a statistically insignificant trend of 3.61 mm over 10 years)
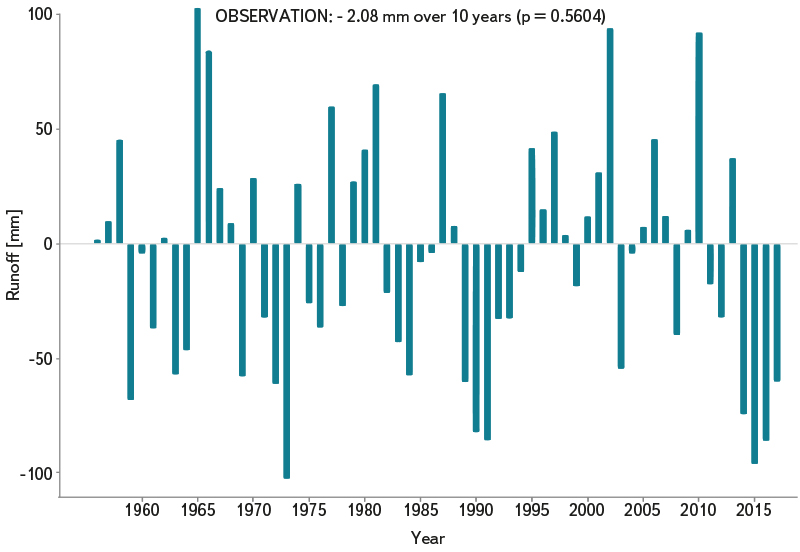
Fig. 3. Mean annual runoff differences (from normal) for the period of 1955–2017 (a statistically insignificant trend of -2.08 mm over 10 years)
Climate Change Scenarios in Water Management
The increment method is used as a standard for calculating climate change scenarios in the context of predicting changes of hydrological balance in the Czech Republic, especially for studies with monthly time steps. This method is based on a transformation of observed data so that changes of transformed variables would correspond to changes derived from climate model simulations. The monthly time step usually considers changes of mean monthly precipitation totals and mean monthly temperature. The daily time step needs to consider also variability changes of variables. Therefore, the ADC (Advanced Delta Change) increment method has been used for calculating climate change scenarios. The increment method is based on a transformation of observed data in a way that ensures that changes between the transformed and original series are the same as changes derived from a regional climate model. Especially in the daily time step, it is desirable that considered transformations regarding precipitation and temperature take into account changes both in the mean and in the variability. To put it simply, this means that extremes may change differently from the mean. When precipitation changes are derived from the climate model, the ADC method takes into consideration also systematic simulation errors. Since temperature is transformed linearly, a systematic error does not have an impact on the resulting temperature transformation [4].
The chosen method has been used to transform selected [5] Global Circulation Models (GCMs) for sub-catchment areas, namely:
- NorESM1-M +
- MPI-ESM-LR + HadGEM2-ES +
- GISS-E2-H + MRI-ESM1 +
- CanESM2 + GFDL-CM3.
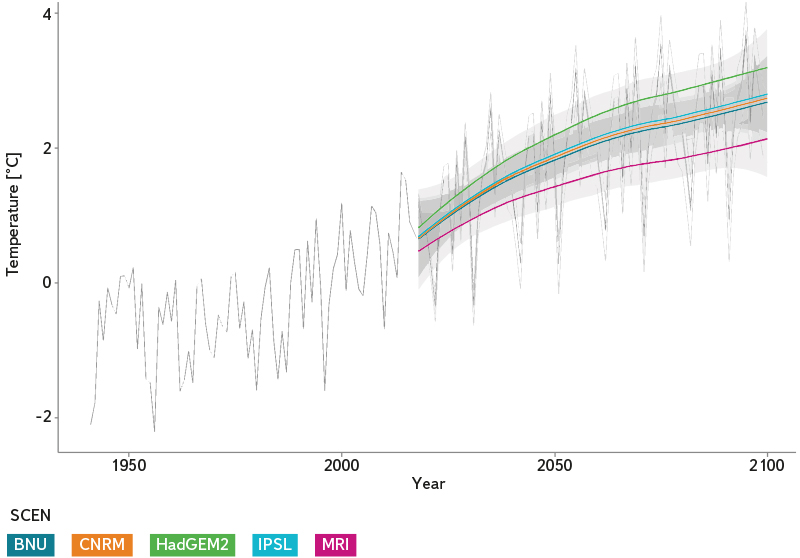
Fig. 4. Air temperature changes according to individual GCMs and RCP8.5
The first model (NorESM1-M) represents a median of the ensemble of all GCMs. The MPI-ESM-LR + HadGEM2-ES models act as controlling GCMs for several Euro-CORDEX RCM simulations. This is after all true also for the selected median model, which also controls one of the Euro-CORDEX RCMs. The GISS-E2-H + MRI-ESM1 models ensure meeting of the condition to cover inter-model variability and the CanESM2 + GFDL-CM3 models enable the last stated condition for the selection to be met. These models have been further tested for water management. Selected RCM models have also been tested. The HadGEM2-ES model has been chosen for the actual testing, which has thus been recommended in studies [6], recommending a median scenario of climate change impacts in water management. Fig. 4 presents temperature development pursuant to RCP8.51.
The following scenarios have been selected to evaluate the water management balance:
1. 0 – current conditions
2. 2 – current climate + 2 °C
3. HadGEM2 – climate based on the outputs of GCM HadGEM2-ES RCP4.5
Modelling of Hydrological Balance
The Bilan model, which is being developed for over 15 years at the Hydrology Department of the T. G. Masaryk Water Research Institute, has been used for modelling hydrological balance. The model calculates in daily or monthly time steps chronological hydrological balance of a catchment area or of a territory. It expresses fundamental balance relations on the surface of the catchment area, in the aeration zone that also includes the vegetation cover of the catchment area, and in the groundwater zone. Air temperature is used as an energy balance indicator that has a significant impact on hydrological balance. The calculation models potential evapotranspiration, actual evaporation, infiltration into the aeration zone, seepage through this zone, snow water equivalent, water storage in the soil and groundwater storage. Runoff is modelled as a sum of three components: two components of direct runoff (that includes also hypodermic runoff) and base flow [7, 8, 9]. The monthly version of the model has been used for modelling hydrological balance.
Hydrological Modelling of Climate Changes
The procedure for modelling the climate change impact on the hydrological regime (see Fig. 5) may be concisely summarised as follows:
1. The chosen hydrological model is calibrated for selected catchment areas using observed data. The hydrological model should have a physical basis to make sure that it yields physically acceptable results also for unobserved conditions.
2. Input variables from a global or embedded regional climate model are transformed to scenario series for the individual catchment areas, namely by:
a.statistical downscaling
b.post-processing of the climate model output, i.e. by using the increment method or correction of systematic errors
It is often necessary to relate the data from calculation cells of the climate model to the centre of a given catchment area by spatial interpolation. It is essential to have observed data at one’s disposal in order to use all methods (a–b) correctly.
3. Simulation of hydrological balance for the scenario period is done using a calibrated hydrological model and scenario series.
4. Modelled discharge for the present and future periods are adjusted in the individual months using the quantile method [10].
Water Balance
The above evaluation of the climate change impact on hydrological characteristics has been followed by an assessment of the security of water demand with the help of the water balance method and simulation modelling of the storage function of water resources and supply systems. The applied method is described in detail [11] as well as the software used [12]. The simulation model simulates behaviour of the system in a chronological series of discrete time steps (the monthly time step has been selected in this case) based on the knowledge of time series of natural discharges (unaffected by regulation and water abstraction/release), requirements for water use (water demand in this case) and maintenance of minimum discharges, technical parameters of the respective elements of the system (volume of the storage space of water reservoirs and water transfer capacity) and a model containing introduced rules of runoff regulation (handling rules).Time series of simulated activities are an output of the simulation: discharge and evaporation from the surface of water reservoirs, water demand, runoff from water reservoirs, water volume and water levels in the storage space of reservoirs. These time series are subsequently statistically evaluated. Security according to the duration of pt, defined in [13] has been evaluated as fundamental characteristics that expresses the security of water demand (to put it simply, it expresses a percentage share of the duration of a period during which water demand and minimum discharge requirements are secured out of the whole duration of the total assessed period.)
Alternatives have been prepared for the above scenarios that describe current conditions and the climate change impact. The aforementioned modelling of hydrological balance has yielded time series of natural discharges and evaporation in the profiles of water reservoirs and water abstraction sites. The overall length of these series has been 718 months (i.e. less than 60 years). Water demand requirements have been assessed alternatively as water demand currently in place and as permitted annual demand values. Maximum annual water demand recorded for compiling the water balance between 2014 and 2019 has been considered as current demand, evenly distributed throughout the year [1]. Permitted demand values, minimum discharge requirements downstream from water reservoirs and storage space volume of water reservoirs have been taken from data contained in the above records. Impact of potential other actual demand and water release in the catchment area of water reservoirs as well as further relevant requirements for the security of water demand for the industry (e.g. water reservoirs Slezská Harta, Kružberk, Morávka and Šance) or agriculture (e.g. water reservoirs Vír I, Vranov and Znojmo). The security of requirements for drinking water demand has always (i.e. in each time step of the simulation) been considered as a priority compared to other requirements for water resources, including requirements for minimum discharges downstream from the water reservoirs. Due to the fact that a potential future situation has been modelled, current operating rules for water reservoirs have not been considered (they have been set with the existing hydrological conditions in mind and a change of these conditions would necessitate their review and optimisation).
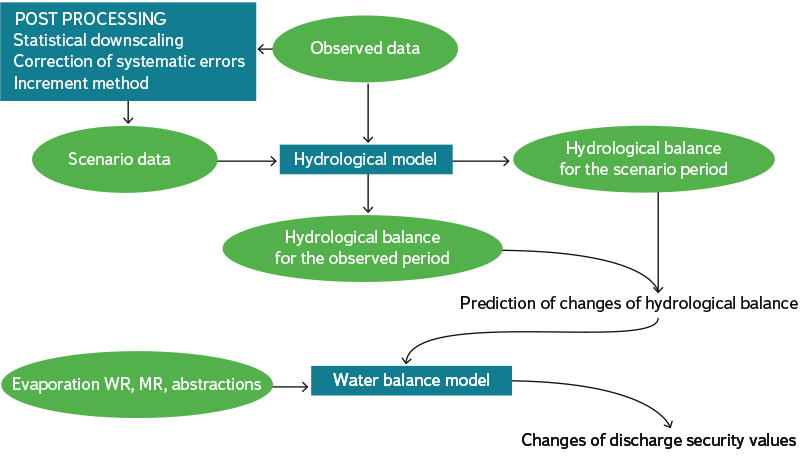
Fig. 5. Scheme of hydrological modelling of climate change impacts
Results
The aforementioned procedure has resulted in a quantification of potential climate change impacts on hydrological characteristics (discharges and evaporation from the water surface and evapotranspiration of the landscape) and subsequent evaluation of the security drinking water demand provided for by water reservoirs in these conditions. Fig. 6 presents changes of natural runoffs (scenario/the present) for scenario 2 and for the HadGEM scenario in the form of a boxplot2.
Results of the evaluation of the security of drinking water demand are presented in Tab. 1 and are illustrated in Fig. 7 by the security value according to the duration of pt. In order to make the presentation more straightforward, water demand has been divided according to achieved security into four groups and has been colour-marked in the following way: (a) the blue colour marks water demand with trouble-free (i.e. pt = 99,9) security of current and permitted demand in all evaluated scenarios; (b) the green colour marks demand with a medium risk with trouble-free security only of current demand in all evaluated scenarios; (c) the yellow colour marks demand with trouble-free security of current demand only in the more favourable HadGEM2 scenario of the climate change impact and (d) the red colour marks demand with a high risk, i.e. where trouble-free security is not ensured in any of the scenarios of the climate change impact.
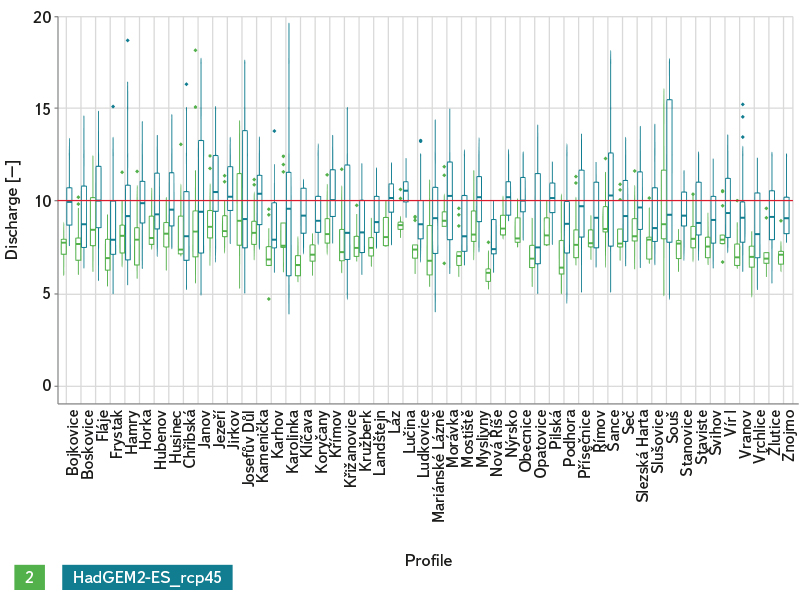
Fig. 6. Reduction in runoff from the catchment area of water reservoirs
Tab. 1. Security of drinking water demand in climate change conditions
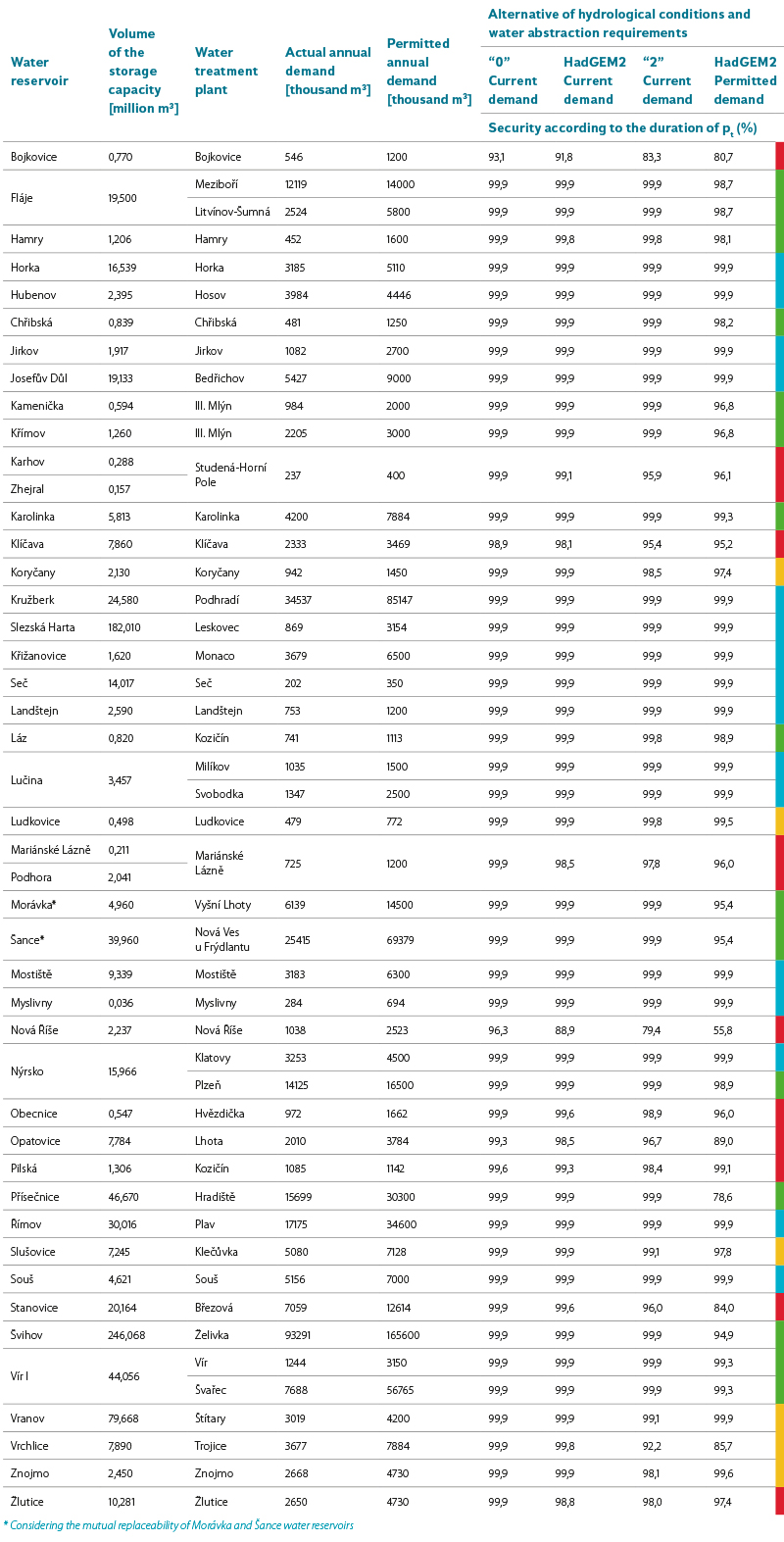
* Considering the mutual replaceability of Morávka and Šance water reservoirs
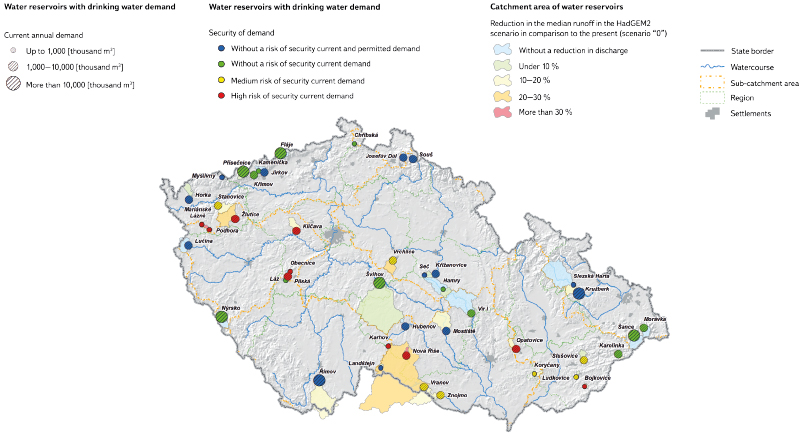
Fig. 7. Risk of insufficient security of requirements for drinking water demand in climate change conditions
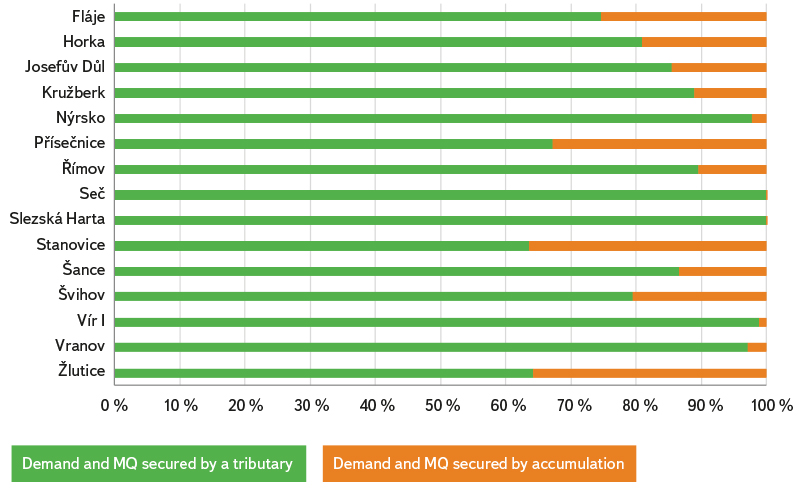
Fig. 8. Use of water accumulation in the storage space of water reservoirs for the security of current water demand and minimum discharges in the HadGEM2 scenario
The following water reservoirs have been evaluated as having a high risk with regard to the security of water demand in climate change conditions: Stanovice, Žlutice, Pilská, Obecnice, Opatovice, Bojkovice, Mariánské Lázně – Podhora, Klíčava and Nová Říše. Medium risk has been evaluated in water reservoirs Vranov – Znojmo, Vrchlice, Slušovice, Koryčany and Ludkovice. Problem-free security of current and permitted water demand has been evaluated in water reservoirs Římov, Nýrsko, Horka, Lučina, Slezská Harta – Kružberk, Seč – Křižanovice, Jirkov, Myslivny, Josefův Důl, Souš, Mostiště, Hubenov and Landštejn.
Fig. 8 illustrates the impact of using water accumulation in the storage space of water reservoirs on the security of current requirements for drinking water demand and minimum discharges in water courses downstream from water reservoirs. A share of the volume of these requirements has been expressed that may be ensured only by an inflow into a water reservoir as well as a share that may be secured only with the help of water accumulation in the storage space. The whole length of the time series of the simulation has been evaluated for water reservoirs with a storage space volume over 10 mil. m3. With regard to simulation of the storage function in the monthly time step (when mean monthly discharges are considered), the impact of discharge variability in the course of individual months has been disregarded.
Discussion
The results of assessing potential climate change impacts on the availability of water resources are naturally burdened with some degree of uncertainty. Uncertainty of prediction of climate change impacts on discharges has been reflected by choosing multiple possible scenarios for hydrological modelling, initial and marginal climate model conditions and their structure. Other uncertainties include selecting the correction of systematic errors of climate models and structure of the hydrological model (modelling by means of various hydrological models). Studies comparing the share of individual sources of uncertainty on the overall uncertainty in hydrological modelling shows that uncertainty that stems from future climate modelling (model structure) significantly exceeds uncertainty related to selecting an emission scenario or uncertainty that follows from hydrological modelling. The overall uncertainty reflected in the result of hydrological modelling is considerable.
Future changes of water demand requirements or the security of minimum discharges represent some uncertainty too (for instance also with regard to potential climate change impacts on water quality). Numerous assessed water reservoirs and water demands are part of water supply systems. Potential deficits in certain water reservoirs may therefore be met by other available water resources in the system; however, on the contrary a requirement may arise to increase water demand from water reservoirs to meet a deficit of other more vulnerable resources in the system (abstraction directly from watercourses or groundwater abstraction). Alternatives have been elaborated for current actual demands and permitted demands. The low share of using permitted values (see Tab. 1) is reflected in the results to a considerable extent. The evaluated low security of permitted water abstraction values in some water reservoirs may be limiting in the future for potential connection of other customers to these resources.
Conclusion
The aim of the above analysis has been to identify potential problems caused by climate change impacts in the timeframe of the year 2050 with respect to drinking water supply provided for by water reservoirs. Hydrological and water management balance procedures have been applied, including modelling of the storage function of water management systems. A potential climate change impact on hydrological characteristics (especially discharges) has been quantified by the applied models, and the security of drinking water demand provided for by water reservoirs has been subsequently evaluated. According to the security that has resulted from the above, the evaluated sites (water reservoirs and water abstraction sites) may be roughly divided into several groups. Out of a total of 45 evaluated water reservoirs, 15 have been evaluated as problem-free for all climate change scenarios as well as for actual demand and permitted water abstraction (among others Římov, Kružberk – Slezská Harta, Horka from the larger water reservoirs); in 13 other water reservoirs a problem-free security has been evaluated in both climate change scenarios only for current demand levels (among others Švihov, Nýrsko, Vír I, Přísečnice, Fláje and Šance). On the contrary, a medium risk of insufficient security of water demand has been identified in 7 water reservoirs (among others Vranov – Znojmo and Stanovice) and a high risk has been established in 10 water reservoirs (among others Žlutice). Prediction of the security of water demand in climate change conditions is naturally burdened with uncertainties both with regard to the necessary simplification of a complex issue in the modelling as well as with regard to further development concerning requirements for water demand (including its functioning within larger water supply systems) and the security of minimum discharges.
Acknowledgements
The study has been elaborated on the basis of a research conducted within No. VI20192022159 “Water Management and Water Supply Systems and Preventive Measures to Reduce Risks for Drinking Water Supply” under programme No. BV III/1-VS, which is funded by the Ministry of the Interior of the Czech Republic.